Oxygen FAQ
Up to date, expert answers to frequently asked questions (FAQ) about oxygen supply systems, respiratory care and pulse oximetry written by OCC & collaborators.
Oxygen Supply Systems
Solar is a very good power option. PV = photovoltaic – refers to the parameters by which solar panels harness electricity. Electricity is a major cost in PSA systems, so if it’s free, oxygen is essentially free. However, there are so many factors involved in solar design: latitude, how much sun you have, irradiance, cloud cover – it’s hard to generalize. In many parts of the world, including India and much of Africa, we see solar systems with batteries that have a 3-year payback. Problem is lack of funding.
Some of the challenges/considerations that need to be made when using solar options include:
Size: You need to know the size needed. E.g., if your generator requires 12 kW, solar should be sized 60kW to take care of the needs of the entire system. Solar power does not work 24 hours, depending on where you are, so you need to consider this.
Area: For a 280 LPM system, electricity requirements are 20 kW, and require 120 kW with solar, and 12000 sq foot area. Managing area is a big challenge. If you can manage to allocate a 6000 square-foot area, you can maybe install solar in this area so that at least 50% of energy will be through solar means. There are also companies that can provide space to help. Electricity boards allow you to generate electricity in one place, and you can manage the electricity where you need it. The availability of this set up varies state by state.
Investment: Solar can be costly, but there are companies in India giving solar with a 15-year contract and fixed unit charges. In Bombay and Delhi – electricity rates average 9-10 rupees per unit, but with solar you can achieve 6, 6.5 rupees/unit – so it’s cheaper.
If you are using solar then it should be 5 times the actual electricity requirement of a generator.
FAQ by Assist International
Yes, they can and often do, this is not a problem. A PSA plant should be designed and built according to an assessment of how much oxygen is needed to supply a direct pipe system plus how much oxygen should be produced to fill cylinders, simultaneously.
FAQ by Assist International
PSA plants need to be operated and maintained safely just like any other industrial motor and machinery, but they are not inherently explosive or combustible. The electrical system must be installed properly and safely. However, oxygen is an accelerant for combustion (fire). So, if there is an open flame or ignition source, an enriched oxygen atmosphere can highly accelerate fire. If oxygen is being exhausted even around the plant, there should be no ignition sources, no open flame, no smoking, no sparks allowed. If high pressure oxygen cylinders are being filled, there are additional safety considerations. NOTE: Electricity requirements are pertaining to the model and specification of each individual PSA plant, this specification must be provided by the PSA manufacturer.
FAQ by Assist International
The practice of ‘bleed-in’ (or adding in) oxygen directly into a ventilator circuit is considered ‘off-label’ and not endorsed by OCC or by most ventilator manufacturers due to significant potential safety concerns. Anecdotally, the practice is commonly reported in some settings. Safety concerns and considerations include:
- Adding flow to the circuit in a volume control mode may cause increased PEEP or inspiratory pressures, alter delivered tidal volume and/or reported tidal volume, and impact sensing/control of pressures (For example at 10 Lpm = 10,000 ml/min –> 167 ml/sec –> so inspiratory time of 1 sec adds 150mL + to set VT). Without precise control of tidal volumes and pressure, there is risk for barotrauma and volutrauma, and delivery of lung protective ventilation will be challenging. Some of these challenges may be mitigated by using only pressure modes of ventilation when using bleed in oxygen supply
- Connections to facilitate this setup are often makeshift and may not be secure; inadvertent disconnect from the circuit or from the flowmeter could result in ventilation/oxygenation failure (all ventilator setups should be tested on test lungs prior to use on a patient)
- Bleed-in may impact ability to sense the patient’s breath triggering
- Bleed-in gas lacks humidity and may dry out secretions further depending on where bleed-in occurs – i.e. bleed-in between the ventilator and the HME or HMEF would in theory mitigate this.
- Bleed-in at the outlet of the heated humidifier or outlet of the ventilator may be advantageous over bleed in at the Wye, because during expiration the inspiratory limb can then act like a reservoir filling with gas and helping increase FiO2
- Bleeding into the inspiratory limb rather than between the HMEF and ETT also mitigates risk to HCWs should the bleed-in O2 tubing become disconnected
- Depending on bias flow and flow rate, during expiration, the gas is flowing out the exhalation valve into the room, and may contribute to wasted gas supply
- Introducing a gas source into the circuit may also introduce a new source for infectious particle exposure to the ventilator or the patient (if not passing first through an adequate filter)
Ventilator connections vary but NIST connectors are common. Most ventilators are supplied with a high pressure hose with a NIST fitting on one or both sides and the other end of the hose with a fitting suitable to match the oxygen source (e.g. Shrader quick release)
- Without access to an oxygen analyser it can be difficult to determine the gas contents in a cylinder. International standards for color of cylinders are not straightforward.
- Most cylinders are filled using pressure swing adsorption (PSA) oxygen plants and thus have a maximum FiO2 of 95% and often considerably less.
- Older oxygen cylinders may be black with a white top. Outside of these standards many variations also exist. All the cylinders in the images below contained oxygen.
- Oxygen analysers should be used to determine gases before use. Cylinders require routine testing and cleaning by certified technicians. Outlet fittings should be examined for damage or corrosion.
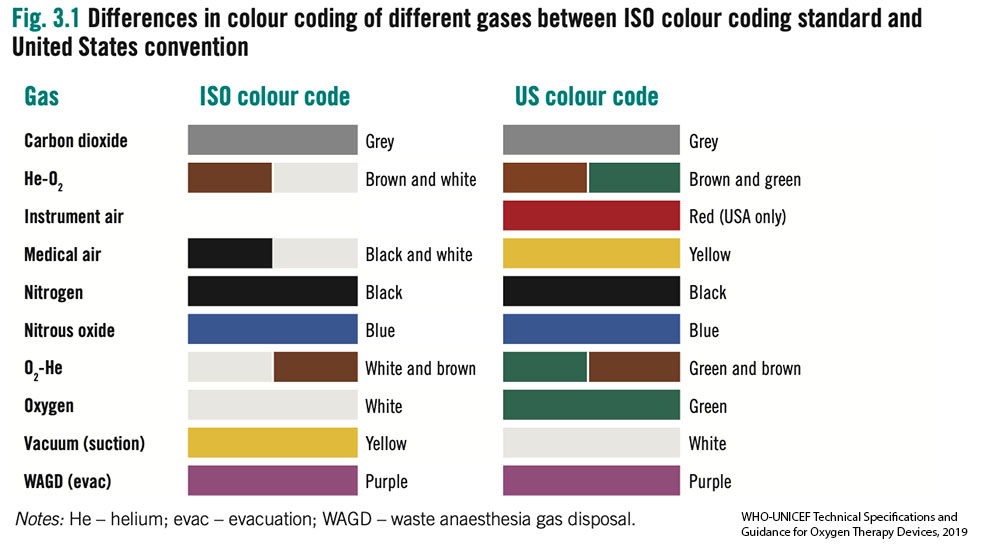
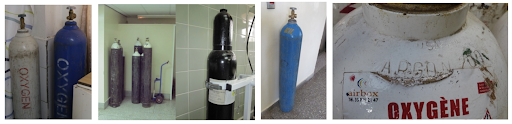
Most oxygen regulators will have a gauge attached, a full J size oxygen cylinder full will have a weight of 78kg (172lb) the oxygen contained in the cylinder weighs approximately 9kg (20)lb, Therefore gauging the volume of oxygen in a cylinder is difficult.
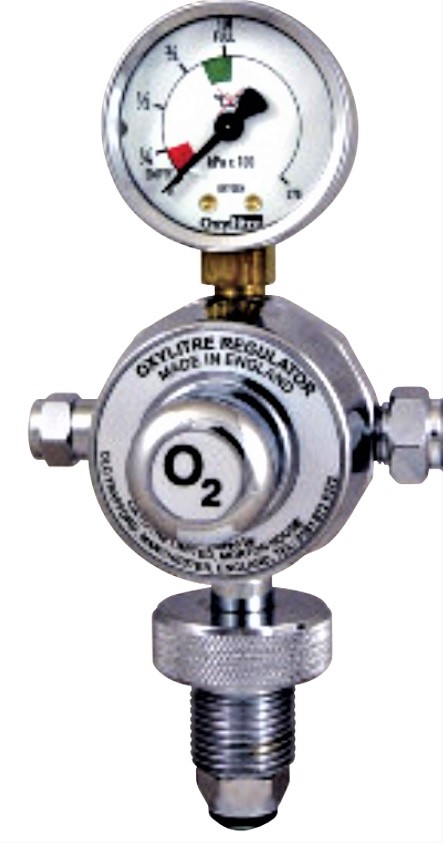
This depends on if the source is liquid or PSA. Plant manufacturers are required to provide and meet certified quality standards and the machine will indicate the purity level during production. If the device is new, you should request the quality certificates. Please download the WHO generic specifications for PSA plants (See our supplementary resources). For periodic checkups, you will need oxygen analyzers.
All devices, including medical devices, should have a quality assurance process that is related also to the documents that the manufacturer provides, or can provide you, from the manufacturing site. We have published the WHO technical specifications that help to have a generic idea of what the standards and certifications are that you need to have in the output of the oxygen generator plants. If the plants are already in style and working, and you want to monitor the oxygen purity…normally in the control panel you will be able to look at what the purity is that they are giving to you. From there you could troubleshoot accordingly. You may also ask the manufacturer or authorized distributor to provide you with specific oxygen analyzers that can help you do the required maintenance and change the filters.
- Provided by Assist International
How do I know how much oxygen my facility is using?
There are multiple strategies to quantify oxygen consumption and need for a facility. The optimal strategy may vary by facility size, oxygen source and intended use of the data. Below are several potential strategies for quantifying oxygen consumption broken down by supply side and demand side approaches.
Of note, if using data to forecast oxygen needs, plan for surges, or expand facility capacity, then current oxygen consumption may grossly underestimate optimal consumption (i.e. you must also account for patients and potential patients who currently do not have access to oxygen due to inadequate current supply).
Supply side indicators:
Measuring facility oxygen consumption may (in some settings) be most accurately done by measuring production of oxygen at the facility source. This may not always be feasible depending on the availability of special equipment and/or human resources to log such data.
- Cylinders – logs (including number, pressure and size) of cylinders delivered to wards and/or delivered to the facility
- Portable oxygen concentrators – logs of use (hours of operation can be accessed from built in hour meter found on most units, though flow rates must be known or can be estimated)
- LOX – logs of cryogenic cylinders filled and emptied based on onsite biomed logs or LOX provider delivery logs; Log of changes in mass of LOX cryogenic storage tank contents may also be available
- Oxygen PSA Plants – monitoring output of PSA plants may depend on how the plant is used – i.e. filling cylinders (in which a cylinder log may be most useful) vs directly supplying a reservoir and/or the facility via piping. Some plants may continuously display hourly flow rate of output which may be logged onsite. An alternative method for PSA plants that directly supply a facility via piping is to record flow with a mass flow meter (Example of mass flow meter) which can be configured to display and save/send such data automatically or detect flow problems.
Quantifiable demand (patient) side indicators:
Quantifying oxygen consumption from the demand side can be challenging and time consuming. This may be the only option when supply side estimates are not available or when you sek to estimate consumption for specific locations within the hospital (e.g. the intensive care unit) or specific patient populations (e.g. COVID19 patients).
When measuring or estimating oxygen consumption, one must attempt to account for leak in the O2 infrastructure as a common source of underestimating oxygen demand. This may occur for many reasons including: human error (e.g. inadvertently leaving an unused flow meter on), suboptimal practice patterns (e.g. titrating to too high of an SpO2 target) or inadequate equipment or maintenance (e.g. poorly fitted regulators, cracked tubing or piping). (Top 10 ways to conserve oxygen)
- Crude modeling of oxygen consumption by patients can be done by using oxygen consumption modeling tools but yields imprecise results.
- Pragmatic primary data collection can be done by recording (at the bedside or via electronic medical record) current consumption per patient in a facility. This includes oxygen delivery device type and settings. While total consumption will vary throughout each day for each patient and thus for the facility as a whole, it may be practical to sample such data once per day or less to obtain snapshots of facility oxygen consumption (Oxygen quantification tools compilation)
- Comprehensive primary data collection can be done daily or multiple times per day to provide the most accurate demand side estimates of oxygen consumption though require considerable resources or comprehensive EMRs.
Common pitfalls in estimating O2 consumption
- Not accounting for leak on the O2 infrastructure
- Inaccurately estimating device consumption (e.g. BIPAP/CPAP leak, ventilator bias flow etc)
- Not accounting for increased flow demand due to decreased oxygen concentration (i.e. a cylinder filled by a low quality supply or a PSA being outstripped of capacity or that is poorly maintained may produced FiO2 that is much lower than expected and thus users may attempt to improve persistent hypoxemia in a patient by increasing flow. Thus, flow consumption may overestimate true demand)
- Estimating only current consumption and not accounting for future increases and decreases in need
Long story short: If you have an oxygen plant that can fill and produce 32 cubic meters per hour, can expect 100-120 cylinders (42L) to be filled in a 24-hour period. Manufacturer gives you rate of capacity if operating every minute, every hour. Assume 80% in real life. Plants are also rated at 1000ft above sea level or lower – much of Sub Saharan Africa is above this so have to derate the plants significantly.
Also, need to consider what size of cylinder you are using – too small, too large cylinders not good – middle is where you get best economy and life span.
Additional details for understanding these conversions:
Cylinder sizes are commonly referred to by volume of liquid (water) such as 42 Liters. Then you must covert the liquid liter size to gas volume. For example, a cylinder that is 42 Liters (liquid) actually contains 6.287 (or round to 6.3) CM of oxygen in a gas state, when the cylinder is filled under pressure to 152 Bar (or 2200 psi).
PSA Plants are built to a specification that identifies a “rated” capacity of production. For example, the plant is rated to produce 16 Cubic Meters per hour. Therefore, you may calculate that 24 hour in a day X 16CM Per hr. = 384CM per day. Once the total CM per day is understood you can divide by the common size of cylinder to know how many cylinders of a given size can be produced per hr or per day. However, it is not likely that the plant will operate every minute of every hour, therefore it is reasonable to assume 80%-90% of the rated capacity will be the actual working capacity. When a PSA plant is ordered, the manufacturer will need to know the location where the plant is to be installed to design the plant with consideration to altitude above sea level, and environmental common temperature range.
FAQ by Assist International
Add the local cost of human resources of plant managers, operators, laborers and security and the operational cost of grid power and/or diesel fuel for the backup generator to the other costs of a 200 LPM capacity plant. The other costs of the 200 LPM oxygen plant cost with cylinder filling capacity will vary by vendor but estimate $200,000 USD. Time to order, deliver and install is approximately 4-6 months depending on the vendor and the supply chain efficiency. Maintenance of spare parts is estimated at $12,000 -$15,000 USD per year, plus service contract at local price. Added cost of infrastructure support for power supply, backup generator power supply, shipping, taxes, and installation cost is based on location and local costs.
FAQ by Assist International
Ventilators without a turbine or compressor generally require both high pressure oxygen (green) and high pressure air (yellow) input to function appropriately, and cannot function at all without at least one of these
Ventilators with a turbine or compressor have the ability to entrain room air directly without a compressed air source; and have variable gas inputs depending on the manufacturer, including the ability to have some combination of:
- low pressure oxygen (e.g. from a portable concentrator), via common smooth bore oxygen tubing (this may require a reservoir to augment FiO2 and an adapter to connect to the device)
- high pressure (55psi/4bar) oxygen (from central pipes or a cylinder)
- high pressure air (usually not, as the turbine or compressor provides this)
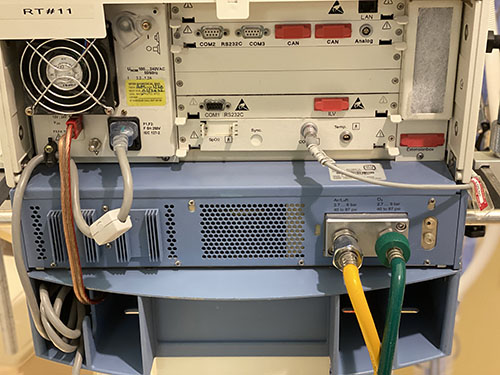
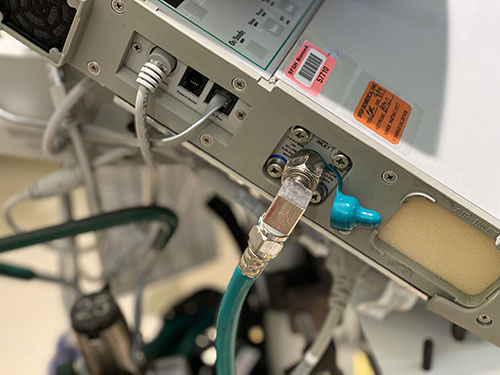
Liquid oxygen is generally more expensive than PSA due to the cost of equipment procurement, infrastructure to support the management, and distribution. After the initial investment for a very large-scale LMO production and distribution system, however, the cost will be diminished over time. LOX, LMO
FAQ by Assist International
First recall the weight of atomic and molecular oxygen: O = 15.99 g/mol and O2 = 32 g/mol
Use the ideal gas law: PV = nRT
P = pressure (in atm); V = volume (in Liters); n = amount of substance (in moles); R = ideal gas constant = 0.0821 (units are (Liters*atm)/(Kelvin*n) ); T = absolute temperature (in Kelvin)
To get the gaseous volume of 1 mole of gas: V=nRT/P
(1 mol)(0.0821 L/atm/K/mol)(273K)/1atm = 22.4 L gas
1 mole gas (STP) = 22.4L = 32g of O2
(32g/mol)(1L/22.4L/mol)= 1.428 g O2 per gaseous liter at STP
Standard temperature and pressure is 1 atm and 273K or O C
If we assume a ‘room temperature’ of 22 degrees C, then:
V=nRT/P
(1 mol)(0.0821 L/atm/K/mol)(295K)/1atm = 24.2 L gas
(32g/mol)(1L/24.2 L/mol) = 1.322 g of O2 per gaseous liter at 22 degrees C
So if you have 1000 L of gaseous oxygen: 1.322 g/L (1000L) = 1.32 kg
The first thing to do is to talk to clinicians in the hospitals and understand their common patient mix, burden of disease, and what they understand to be the demand for oxygen in their setting, whether it be a health clinic or hospital department. Consider this as well though: in some hospitals clinicians may not have had opportunity for adequate training in respiratory care, and after providing clinical training opportunities oxygen consumption may increase due to increased usage of pulse oximeters to diagnose and treat patients. Pulse oximetry also can help to prevent over-oxygenating a patient and conserve oxygen. Therefore, clinical training components are important.
We would also advocate looking at duplex systems – 2 smaller PSA systems than a large plant because compressors do not modulate, and electricity can be 70% the cost of 1 L of oxygen. Moreover, in some hospitals where we have converted from cylinders to piped oxygen, we have seen in some cases a 300% increase in use of oxygen. Note that the direct pipe system does not require a PSA plant at your hospital, it can be supplied by cylinders.
Not everyone is going to have plants at their hospital. Why? If someone has to go a pick up a cylinder- heavy, time consuming, difficult, might actually be neglected altogether. But when piped it is readily available at the patients’ bedside and you just have to hook up a nasal cannula or mask. This means patients are getting low flows of oxygen much earlier and higher flows when they need it. So, your oxygen consumption can often go up. Distribution system also depends on if you are piping filling cylinders, working hours, size, etc.
The tool that we use to calculate this is from the OGSI website, found at this link here: https://www.ogsi.com/product/hospital-systems/. You should check this against what your facility is doing in terms of treatment and clinical services, you may need to adjust accordingly. Here is the formula:
Hospital System Sizing Estimator:
[# of beds x 0.75 liters per minute (LPM)] + [# of other outlets (ICU, Operating Theatre, etc.) x 10 liters per minute (LPM)] = total liters per minute (LPM)
*Liters per minute (LPM) x 2.1 = Standard Cubic Feet per Hour (SCFH)
FAQ by Assist International
The primary consideration should be to ensure that the oxygen system is dependable and sustainable. The best main supply source of oxygen to a patient is a direct piped system that connects to the patient bedside from a PSA plant (or oxygen reservoir supplied by a liquid oxygen source). The direct pipe system is highly recommended and preferred. The alternative is to bring cylinders to the bedside. The back-up supply source for a direct pipe system would be a manifold of cylinders and additional spare cylinders to adequately supply the pipe system if the main supply is temporarily not operating.
FAQ by Assist International
While this does occur, the short answer is ‘usually not.’
Atmospheric air (commonly referred to in clinical settings as ‘room air’) is composed of approximately 78.08% nitrogen, 20.95% oxygen, 0.93% argon, 0.04% carbon dioxide, variable amounts of water and negligible amounts of other gases. Oxygen concentrators use zeolite molecular sieves to separate oxygen from nitrogen in room air and produce a high concentration of oxygen. However, the sieve beds have a similar affinity for argon as they do for oxygen. This results in oxygen concentrators also performing as ‘argon concentrators,’ and the output of both gases increasing by nearly 5-fold in the output from a concentrator (~93-95% oxygen and 5% Argon).
There is no evidence of this concentration of argon (or much higher concentrations) having harmful effects in humans. There are data that argon, similar to some other noble gases (e.g. xenon) may have neuroprotective effects and at very high pressures (beyond seen in the clinical setting) may have some anesthetic properties (PMID: 31470983; PMID: 20472713; PMID: 19500647; PMID: 20236500).
High concentrations of argon will decrease the concentration of oxygen delivered to the patient, and this should be accounted for in the clinical setting. For most clinical settings and scenarios this is unlikely to be clinically significant. This is no different than many of the other gases commonly added to the breathing mixtures (e.g. nitrous oxide, helium, inhaled anesthetics).
The special case of low-flow, fully or partially closed circuit anesthesia is sometimes cited as a potential scenario where build of argon could occur at higher levels and cause potential harm. This has been studied and concluded that oxygen concentrators can be used for oxygen supply in fully or partially closed circuit anesthesia delivery systems for indefinite duration as long as the fresh gas flow (FGF) input flow rate is at least twice the oxygen consumption (e.g. FiO2 0.5 and FGF ~1 LPM), nitrous oxide is not used or is being accounted for, and the system is periodically opened for fully closed systems.
Unsurprisingly and unfortunately, arguments citing argon as a reason to not use oxygen concentrators in clinical care are usually driven by those with commercial interest in liquid oxygen.
References:
There could be many reasons for this. If it is connected to the pipe network, it is possible the design of the pipe was not done correctly. Under “Supplementary Resources”, there are recommendations posted about rational use of oxygen that can provide further detail.
FAQ by Assist International
It depends on many local factors. Consider the materials used locally, how dusty the area is, and how hot the area is. Generally, we prefer to use masonry block and concrete for the walls and concrete floor. For the roof, consider factors such as tropical storm frequency in the area. If the area is subject to tropical storms with high winds, then consider a concrete roof. Also consider thermal mass and/or insulation of roof to minimize heat transmission.
PSA plants are much more expensive if provided in a container (also the time to build is longer). The advantage is ease of installation without a building, but a support building will be needed to manage cylinders that are being filled and the manifold for the cylinder supply (primary supply or backup supply) to a pipe system.
FAQ by Assist International
- Large J size (6800 L) oxygen cylinders (filled at 137bar/~2000psi) commonly have a Bull Nose (BS 341) oxygen outlet. Many smaller cylinders have the same outlet. Pin Index cylinders (ISO 407) may be unavailable in some resource variable settings.
- A regulator with Bull Nose connector attaches to the tank to decrease the pressure from 137bar/2200psi to ~4 bar/50psi
- Regulator outlet connectors vary (4 bar) high pressure connectors are 1/4” hose outlet, 3/8” BSP or Shrader (BS 5682) quick release (left to right in image).
- Check out our image library for oxygen connector types
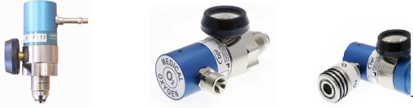
Key points for sustainability of an oxygen supply system begin with identification of a cost analysis for operational expenses:
- Cost of power to operate 24/7 – include cost of grid power and diesel fuel for back-up generators.
- Cost of spare parts package that is specific for the type of equipment to be installed. For example: key equipment for spare parts includes the air compressor, PSA generator and the booster compressor for filling cylinders (if filling cylinders). Budget for the spare parts and confirm the supply chain to order and import the spare parts.
- Staffing costs of Engineers for service and daily operations.
- Cost of service contracts for outside service provider to do regular maintenance
FAQ by Assist International
- Most portable oxygen concentrators use pressure swing adsorption to intake room air and output ~93-96% oxygen
- Most concentrators produce oxygen at 5 or 10LPM max, and at low pressure (this means far less than the 50psi/4bar needed to run HFNC, and most ventilators and NIPPV devices)
- There are a few portable devices capable of higher flow / high pressure output (e.g. 15-30LPM, or 50psi)
- Make sure the FiO2 is adequate (>~82-90%) at the max flow rate, as many concentrators make claims of high flows but deliver limited FiO2 (and thus limited benefit) at those flow rates.
- Performance of concentrators may vary by manufacturer in hot and humid environments (Peel et al, Anaesthesia, 2013)
- Zeolite does not last forever – needs to be replaced around 25,000 hours, though may be considerably less depending on use, maintenance and humidity
- While they can run continuously for long periods of time (months, years) they do require routine maintenance
- When run beyond max flow (flowmeter opened all the way), this can cause premature failure due to excess moisture building up in the sieve bed
Additional Resources:
- How to setup an oxygen concentrator by wikiHow
- Preventative maintenance for O2 concentrators – Med Equipment Troubleshooting – by Engineering World Health
- Frank’s Hospital Workshop Tutorials – Oxygen Concentrators – by Frank Weithoner
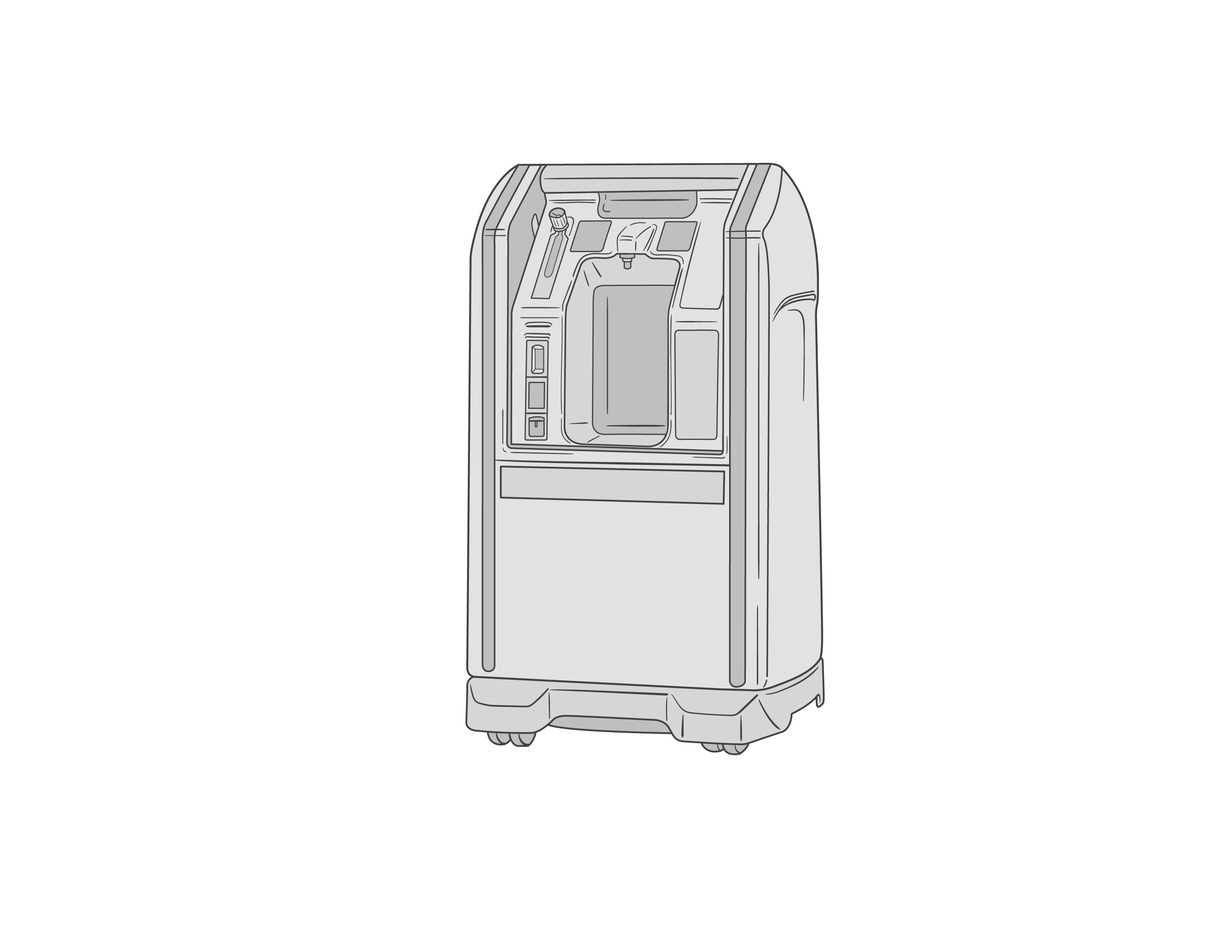
Database for oxygen concentrator specifications
- Supply shortages – Everyone in the world has been trying to buy these since the beginning of the pandemic. Be wary of large stockpiles of ‘available’ devices from unknown manufacturers.
- Flow rates – Concentrators commonly produce 3, 5, 8 or 10 LPM continuous flow output, though some may produce 10 or more LPM. All devices should have an adjustable flowmeter (and if planning to use for pediatric patients, a minimum flow rate of 0.5 LPM may be needed. Make sure the FiO2 is adequate (>~82-90%) at the max flow rate, as many concentrators make claims of high flows but deliver limited FiO2 (and thus limited benefit) at those flow rates.
- For stable patients on stable amounts of oxygen who are using the concentrators in climate controlled rooms (e.g. COPD at home, one of the original use scenarios for portable concentrators), a device with relatively low output < 5LPM may be sufficient
- For hospitalized patients who may have fluctuating needs (e.g. acute respiratory illness, COVID19), concentrators with higher outputs (e.g. 10-15 LPM, or higher) may be more desirable
- Oxygen concentration – WHO Technical Specifications stipulate output of >82% concentration of oxygen at max rated flow rate at 40 degrees C and 95% relative humidity (RH). Most high quality devices should have >90% output. NOTE: many concentrators, especially smaller (<10kg) units only produce 30% FiO2 at max flow rates, which is inadequate to care for most patients with acute respiratory illnesses.
- Power – ensure compatibility with local power supply and outlet adapters; and ensure efficiency <70W/LPM. Many oxygen concentrators do not have backup batteries that will power the device in the event of power failure. Consider using with a voltage stabilizer and surge protector.
- Noise – <50 dBA; many noisy units on a single ward can make it difficult to hear patient alarms
- Durability – Consider IP21 or greater to offer special protection against moisture
- Outlet pressure – must generate at least 55kPa at all flows and ensure stable (narrow range) of output pressure (+/- 1 psi)
- Operating environment – ensure safe operation in 0 to 40 degree C, and RH 15 to 95%; ensure certified to altitude suitable for destination (many are not certified above 3000m due to partial pressure changes)
- Alarms – device must have alarms for low oxygen concentration alarm (<82%), no flow, high/low pressure, low battery, power supply failure, high temperature. NOTE: be cautious of devices with prolonged warm up periods, maximum operating durations (good units should work continuously), or auto-shutoff functions.
- Spare parts – ensure spare parts for one year – including spare battery for power failure alarms (more recent models may use a capacitor and do not have a battery), intake filter, and internal filter
- Delivery devices – don’t forget to ensure access to delivery devices (e.g. nasal cannula, face masks and humidification bottles)
- Access to maintenance tools – ideally facilities should have pressure gauge, FiO2 meter oxygen analyzer, and spare parts for routine maintenance; consider a local service contract or identifying a provider
- Training – recipients of concentrators should be provided with training on operation, troubleshooting and maintenance
- Be wary of devices with auto-shutoff timers as these can increase likelihood for errors
Additional Resources:
- Evaluation of O2 concentrators for use in countries with limited resources
- How to setup an oxygen concentrator by wikiHow
- Preventative maintenance for O2 concentrators – Med Equipment Troubleshooting – by Engineering World Health
- Frank’s Hospital Workshop Tutorials – Oxygen Concentrators – by Frank Weithoner
- WHO Technical Specifications for oxygen concentrators
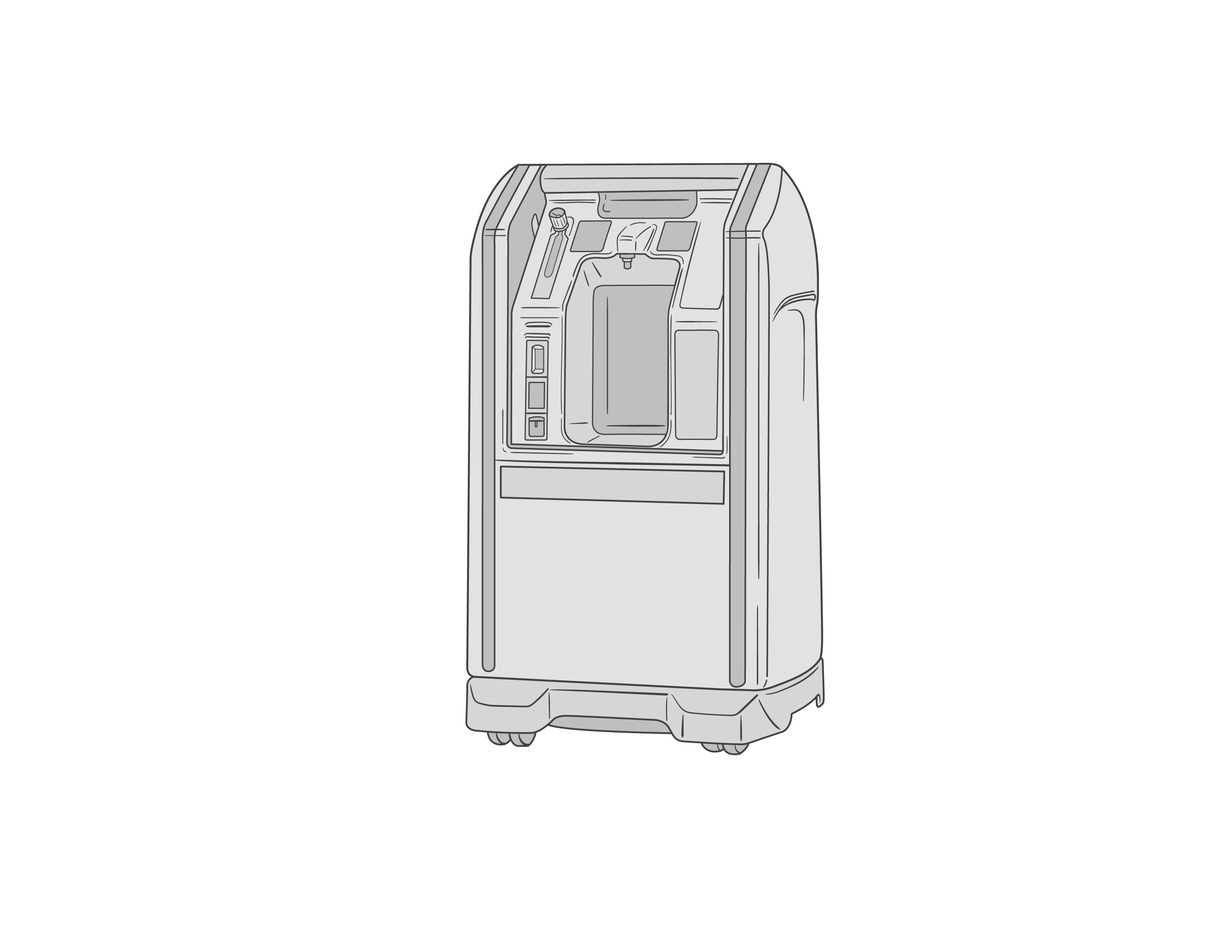
Here are some tips on how to safely conserve oxygen depending on the local context:
- Be cautious with high flow nasal cannula – high flow nasal cannula uses up to 60-70 LPM flow and can deplete stores quickly. This delivery device generally should not be used on cylinders unless a manifold system is present and a large cylinder pool is present. Additionally, even when supply may be ample, multiple HFNC devices in simultaneous use may cause system pressure failure or a rate of decline that causes excess freezing of liquid oxygen sources (which will decrease liquid oxygen output further). Always wean patient flows as quickly as possible and transition to alternative lower flow devices as soon as tolerated. There are some turbine and Venturi based devices which do not require a robust medical air supply, but these devices still consume O2 at high rates.
- Use evidence based SpO2 goals and avoid hyperoxia – Avoiding higher than necessary SpO2 goals will save vast quantities of oxygen (Read more on Optimal SpO2 Goals). The difference in SpO2 goal of 90 vs 92% in COVID19 patients can translate to 2-3x less oxygen utilized. This requires continuous or frequent pulse oximetry checks and careful, frequent titration. (see SpO2 goals). There are multiple auto-titration devices that are receiving increasing attention, but yet to see widespread use.
- Consider oxygen conserving devices – There are multiple types of oxygen conserving devices, which may or may not be useful depending on the use case. These devices include oxymizers (both pendant style and nasal reservoir style) as well as conserving devices that are placed on the cylinder directly (also known as ‘pulse dose’ devices). Reservoir-based devices conserve oxygen by enabling a higher FiO2 with lower flow rate as the patient is breathing some oxygen from the reservoir. These devices were designed to work in patients with chronic lung conditions, and their performance in patients with acute respiratory failure is largely unproven (i.e. if a patient has a higher minute ventilation). Pulse dose devices can be expensive. There are numerous other oxygen delivery devices that claim or prove oxygen conservation, however, for most of these devices performance and outcome data are lacking for acute respiratory failure patients.
- Consider liquid oxygen when possible – this is the most efficient way to store and produce oxygen, based on size:capacity. However, liquid oxygen requires considerable infrastructure on site and regionally(e.g. roads, trucks, oxygen separating plants, etc).
- Utilize additional O2 storage methods – these may be mobile liquid oxygen units, additional cylinders (Size H/J), or reservoir devices.
- Inspect and eliminate O2 system leaks – leaks in oxygen infrastructure and delivery devices are common. Common causes to include:
- Using excess flow
- Not turning off devices that not in use (e.g. anesthesia machines or flowmeters)
- Incorrectly secured fitting from cylinders
- Leaks in high or low pressure tubing due to lack of adequate maintenance
- Utilize oxygen concentrators – Consider utilizing oxygen concentrators to preserve cylinders and central supply when possible. There are multiple ways to split an oxygen concentrator to maximize the benefit of its output for multiple patients.
- Carefully adjust intentional leak for CPAP/NIPPV – properly used NIPPV/CPAP will have a leak. Too much or too little can be problematic depending on the interface and patient. Proper titration requires close monitoring and regular adjustment
- Account for Bias Flow & consider devices without bias flow – Some ventilators utilize continuous flow during the expiratory cycle – ‘bias flow.’ In some cases, this adds considerably to oxygen consumption (see ventilator settings in step 3 of this calculator) above and beyond minute ventilation consumption. See manufacturer specifications and account for this in planning.
- Consider systems that allow on-site filling of cylinder – PSA plants and deployable oxygen concentrator systems (DOCS) can be used to refill cylinders. Specifications vary widely depending on need.
- Frequent inspection and countermeasures to avoid liquid oxygen failure:
- Daily inspection to look for excess icing with increased use and colder weather, liquid oxygen evaporators may freeze – dramatically reducing O2 output. Regular inspection and countermeasures are critical (e.g. de-icing or warming systems to prevent freezing
- Inspect daily for potential leaks
- Maintain daily line pressure log and fill level
- Frequent inspection and countermeasures to avoid portable oxygen concentrator failure:
- Avoid running beyond max flow (this can cause humidity to build up in the zeolite and cause decreased efficiency and failure)
- Inspect and clean air intake filter regularly (weekly)
- Ensure functioning voltage stabilization unit if applicable
- Even when not in use, be sure to periodically turn on the concentrator for 30 minutes weekly – this will flush the system and filters of humidity which can damage the device
- Never run the unit without proper filters in place
- Keep the air intake away from walls and curtains
- Frequent inspection and countermeasures to avoid cylinder failure:
- Keep regulators and valves dust free
- Avoid (fire) ignition sources
- Ensure cylinders are stored and secured properly to prevent falling over
- Always open cylinder valves slowly
- Estimate your burn rate and plan ahead – in some settings oxygen shortages can be avoided or ameliorated by planning ahead. Keeping track of daily facility consumption and modeling surge scenarios can help with planning for production and maintenance (PSA plans), cylinder numbers needed, refill frequency (liquid oxygen). There are many tools to help with planning. The online interactive tool (below) helps quickly model ward scenarios with varying levels of detail.
- Ensure adequate power and backup power for PSA/concentrators – power failures can be a significant and abrupt cause of oxygen supply failure. If dependent on oxygen concentrators (whether portable or PSA plant), it is imperative to:
- Utilize voltage stabilization units where power supply voltage is variable
- Implement and regularly test backup power supply and/or backup cylinders in the event of power failure
Additional Resources:
Oxygen supply and demand calculator by OpenCriticalCare.org
Blakeman TC, Branson RD. Oxygen supplies in disaster management. Respir Care. 2013 Jan;58(1):173-83.
Compilation of oxygen planning tools and presentations by OpenCriticalCare.org
Frank’s Hospital Workshop Tutorials – Oxygen Concentrators – by Frank Weithoner
Oxygen conservation infographic – Branson et al, CHEST, May 2021
PSA is the acronym for “Pressure Swing Adsorption”, which is the name of the process that takes place inside the Oxygen Generator Plant. PSA plants extract oxygen using a molecular sieve, which expels nitrogen and leaves a more purified oxygen product, which is stored in a tank until ready for distribution by a pipe system. The basic technologies of the PSA plant include: the air (feed) compressor, the air dryer, prefilter systems, the PSA generator, and the booster compressor. Review the Assist International webinar “Essential Considerations Related to a PSA Plant” HERE for more details. You may have also heard of “VPSA” – “Vacuum Pressure Swing Adsorption”. For a detailed explanation on VPSA see: https://www.trimechindia.com/blog/oxygen-plants-whats-difference-psa-vpsa-oxygen-plant/
FAQ by Assist International
Most ventilators operate with 35-65 psig/4 bar inlet which is sufficient to operate the blender (i.e. what mixes 100% oxygen and air). Without adequate pressure the device may not function at all or with inaccurate delivery of volume, pressure and oxygen concentration. If a reliable high pressure gas supply is not present, consideration of ventilators with turbines or compressors may be critical.
Some ventilators that measure FiO2 will alarm if you connect something less than 100% oxygen to them. Some ventilators (especially transport ventilators) may not have an FiO2 sensor and just display the blender fraction setting, not a direct measurement of concentration (this requires calibration during preventative maintenance).
High pressure oxygen sources are capable of delivering oxygen at ~50psi/4bar to a device. These include oxygen cylinders (via regulator), oxygen plants via a compressor, liquid oxygen (via vacuum insulated evaporators) and very few portable oxygen concentrators (via additional compressor). High pressure oxygen is required for most ventilators, high flow nasal cannula and non-invasive positive pressure ventilators when taking care of critically ill patients.
Low pressure oxygen sources deliver oxygen at far less than <50psi/4bar. These include oxygen from a low flow flowmeter or a portable oxygen concentrator. Generally, these cannot deliver high enough oxygen concentrations to take care of severely hypoxemic patients.
***Some ventilators (e.g. LTV2200, Zoll 731) can operate with either high pressure or low pressure oxygen input. Other ventilators (e.g. PB560) may only be capable of utilizing a low pressure oxygen input. Of note – when utilizing low pressure oxygen input, some ventilators may not be able to provide high enough concentrations of oxygen to care for critically ill patients. Check manufacturers’ reports for maximum oxygen concentration delivery.
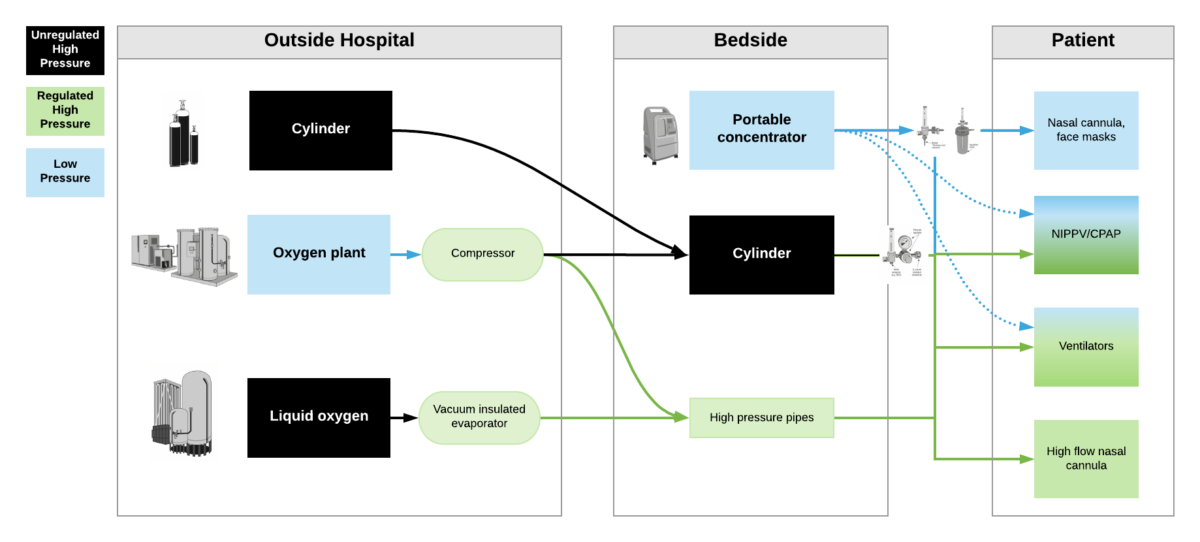
The first exhaust system directly exhausts the large compressor. The second exhaust would exhaust the room and make sure the room has good air circulation and the temperature is moderated. Also, sometimes the air dryer needs exhaust.
FAQ by Assist International
- The short answer is that it depends on many factors and varies by ventilator model (so check the manufacturers’ specifications)
- Some ventilators like the LTV 1200/2200, PB560 and Zoll 731 can run off of a low pressure oxygen supply. They utilize 21% ambient air from the room via a built in compressor or turbine and mix that with the low pressure oxygen input.
- When utilizing low flow oxygen, ventilators usually will not allow you to use the blender – i.e. you can’t set the FiO2
- Concentration of oxygen delivered to the patient (FiO2) is determined by: minute ventilation, flow of oxygen supply into the vent, concentration of oxygen supply into the vent, and potentially other factors.
- For example:
- LTV 1200/2200 – If input flow is set to minute ventilation, then the LTV1200/2200 can deliver ~100% FiO2 up to ~20LPM minute ventilation. For a patient with 10 LPM minute ventilation and 5 or 10LPM input low pressure oxygen, max FiO2 delivered to the patient is approximately 50 and 75% respectively. (See Figure below left)
- Zoll 731 – If input flow is set to minute ventilation, and an external reservoir (e.g. ZOLL 731) is used on the compressor intake, then FiO2 of ~100% is possible on the Zoll 731 for minute ventilation up to ~20 LPM. (See Figure below, bottom) For more information on using the ZOLL 731 with low pressure O2 sources see the users manual and this quick reference.
- PB560 – Some ventilators like the PB560 that only accept low flow oxygen may be limited in the amount that can be delivered into the device at low pressure and thus be limited in FiO2 delivery to the patient (e.g. the PB 560 is designed to deliver a percentage of oxygen equal or lower than 50%. Exceeding this value may cause the ventilator to malfunction and put the patient at risk). (See Figure below right)
- Of note, 50% FiO2 is approximately equivalent to that delivered by a simple facemask at 10LPM (Read more about FiO2 delivered by various oxygen delivery devices)
- When using low pressure oxygen supply for devices with bias flow (flow during the expiratory time) and/or during NIV modes with leak compensation, the FiO2 can be diluted significantly.
- When using low pressure oxygen supply, you likely will need to change the ventilator setting to indicate such a source is being used (e.g. ‘reservoir mode’ or ‘low pressure input’)
- Due to slight difference in the densities of air and O2, tidal volume may decrease slightly as FiO2 increases via low pressure entrainment. See manufacturer’s specifications, though generally this would be expected to be less than 10% change in tidal volumes.
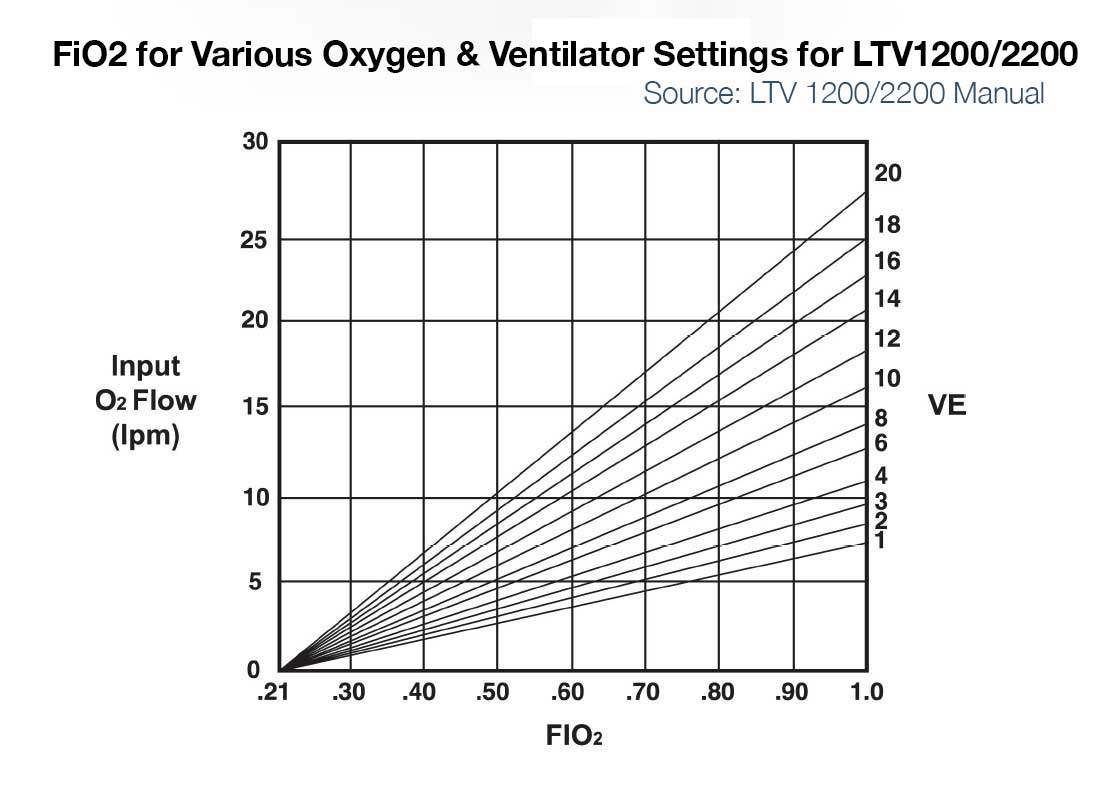
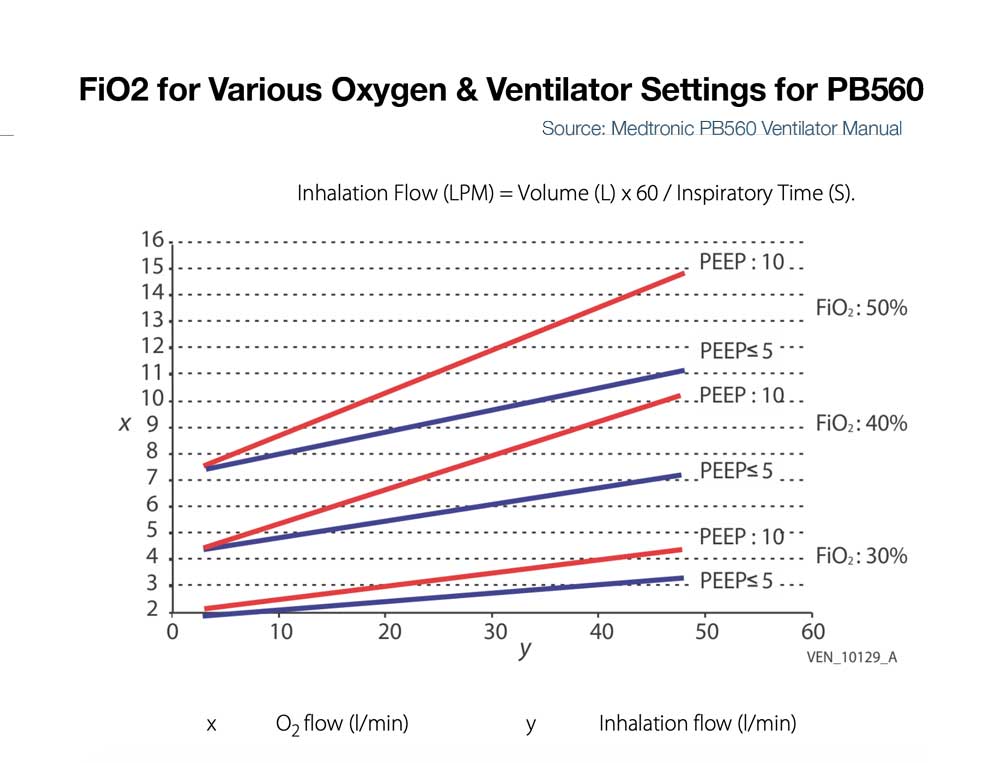
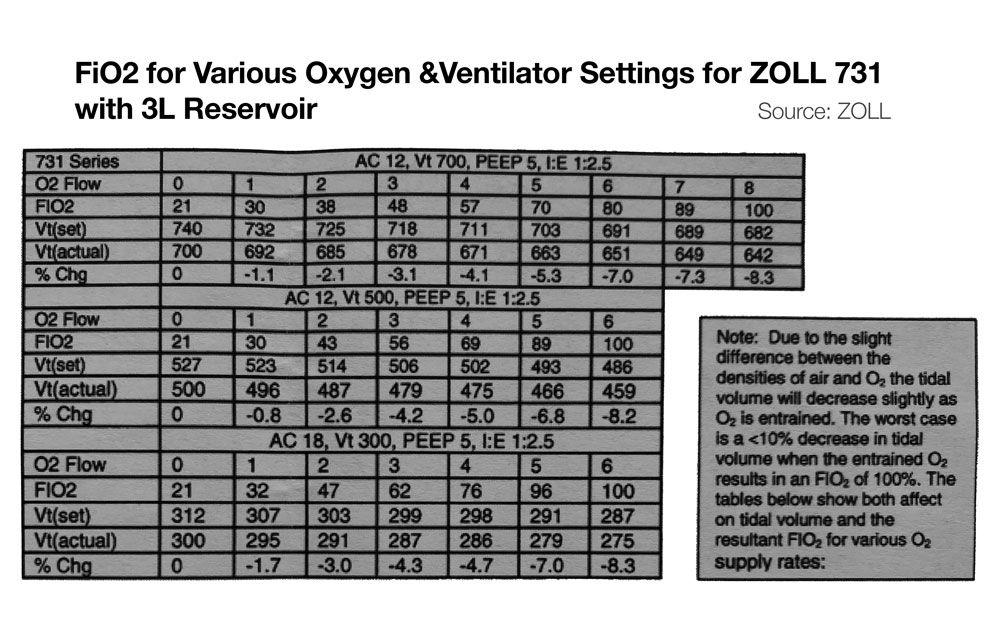
For staff qualifications, first determine if the staff is expected to simply operate the plant or if the staff is also expected to provide maintenance-service for the plant.
If the staff is expected to simply operate the plant, then the minimum qualification of the should be 1 staff that is a certified/qualified electrical, mechanical, or biomedical technician, plus if cylinders are to be filled, then add staff of 1 or 2 basic laborers to handle cylinders (the number of added laborers needed will depends on the number of cylinders to manage).
If the staff is expected to perform basic and/or advanced maintenance/service to the PSA plant then a certified electrical, mechanical, or biomedical engineer should be added to the staff.
The total number of staff to be hired should be based on the plan of operational hours of the plant. For example, a PSA plant is typically planned to operate for 24 hours per day, 7 days per week, therefore the total number of staff to be hired should be planned accordingly.
Initial Basic Training for PSA plant technicians and engineers is recommended to begin at the time of plant installation and commissioning by the manufacturer’s installation team. The manufacture should provide complete instruction regarding how to operate the plant, monitor, understand and record the proper performance parameters of the plant, instruction of simple and routine maintenance for the plant according to manufacturer’s specification, basic trouble-shooting, and standard operating procedures for acquiring support for service and maintenance when needed.
Advanced levels of service should only be performed by competent trained engineers and technicians, and this usually means that an “outside” service contract will be required to provide the advance levels of service that will be needed. Overall, the availability of advanced level training for PSA services is scarce and will require the PSA owner to prioritize and finance efforts to locate training opportunities to increase the knowledge, skill and abilities of their own technicians and engineers.
FAQ by Assist International
Public private partnerships are new and often, public procurement laws are geared towards buying and owning things- not getting services which is what PPPs are all about. The earliest PPPs, and maybe the most developed, in the UK were very much geared towards extremely large infrastructure projects so if you are looking at how to do PPPs, most likely you will get information or the tools on how to do a bridge, a port, an airport, or something like that. Services have not been the major concern of most of these PPPs and you will find that there is just not much experience. So, to do a successful PPP, it requires a lot of education or capacity-building for governments for them to understand what you are trying to do and to demonstrate the benefits.
FAQ by Assist International