Oxygen FAQ
Up to date, expert answers to frequently asked questions (FAQ) about oxygen supply systems, respiratory care and pulse oximetry written by OCC & collaborators.
Portable oxygen concentrators
How do I know how much oxygen my facility is using?
There are multiple strategies to quantify oxygen consumption and need for a facility. The optimal strategy may vary by facility size, oxygen source and intended use of the data. Below are several potential strategies for quantifying oxygen consumption broken down by supply side and demand side approaches.
Of note, if using data to forecast oxygen needs, plan for surges, or expand facility capacity, then current oxygen consumption may grossly underestimate optimal consumption (i.e. you must also account for patients and potential patients who currently do not have access to oxygen due to inadequate current supply).
Supply side indicators:
Measuring facility oxygen consumption may (in some settings) be most accurately done by measuring production of oxygen at the facility source. This may not always be feasible depending on the availability of special equipment and/or human resources to log such data.
- Cylinders – logs (including number, pressure and size) of cylinders delivered to wards and/or delivered to the facility
- Portable oxygen concentrators – logs of use (hours of operation can be accessed from built in hour meter found on most units, though flow rates must be known or can be estimated)
- LOX – logs of cryogenic cylinders filled and emptied based on onsite biomed logs or LOX provider delivery logs; Log of changes in mass of LOX cryogenic storage tank contents may also be available
- Oxygen PSA Plants – monitoring output of PSA plants may depend on how the plant is used – i.e. filling cylinders (in which a cylinder log may be most useful) vs directly supplying a reservoir and/or the facility via piping. Some plants may continuously display hourly flow rate of output which may be logged onsite. An alternative method for PSA plants that directly supply a facility via piping is to record flow with a mass flow meter (Example of mass flow meter) which can be configured to display and save/send such data automatically or detect flow problems.
Quantifiable demand (patient) side indicators:
Quantifying oxygen consumption from the demand side can be challenging and time consuming. This may be the only option when supply side estimates are not available or when you sek to estimate consumption for specific locations within the hospital (e.g. the intensive care unit) or specific patient populations (e.g. COVID19 patients).
When measuring or estimating oxygen consumption, one must attempt to account for leak in the O2 infrastructure as a common source of underestimating oxygen demand. This may occur for many reasons including: human error (e.g. inadvertently leaving an unused flow meter on), suboptimal practice patterns (e.g. titrating to too high of an SpO2 target) or inadequate equipment or maintenance (e.g. poorly fitted regulators, cracked tubing or piping). (Top 10 ways to conserve oxygen)
- Crude modeling of oxygen consumption by patients can be done by using oxygen consumption modeling tools but yields imprecise results.
- Pragmatic primary data collection can be done by recording (at the bedside or via electronic medical record) current consumption per patient in a facility. This includes oxygen delivery device type and settings. While total consumption will vary throughout each day for each patient and thus for the facility as a whole, it may be practical to sample such data once per day or less to obtain snapshots of facility oxygen consumption (Oxygen quantification tools compilation)
- Comprehensive primary data collection can be done daily or multiple times per day to provide the most accurate demand side estimates of oxygen consumption though require considerable resources or comprehensive EMRs.
Common pitfalls in estimating O2 consumption
- Not accounting for leak on the O2 infrastructure
- Inaccurately estimating device consumption (e.g. BIPAP/CPAP leak, ventilator bias flow etc)
- Not accounting for increased flow demand due to decreased oxygen concentration (i.e. a cylinder filled by a low quality supply or a PSA being outstripped of capacity or that is poorly maintained may produced FiO2 that is much lower than expected and thus users may attempt to improve persistent hypoxemia in a patient by increasing flow. Thus, flow consumption may overestimate true demand)
- Estimating only current consumption and not accounting for future increases and decreases in need
Ventilators without a turbine or compressor generally require both high pressure oxygen (green) and high pressure air (yellow) input to function appropriately, and cannot function at all without at least one of these
Ventilators with a turbine or compressor have the ability to entrain room air directly without a compressed air source; and have variable gas inputs depending on the manufacturer, including the ability to have some combination of:
- low pressure oxygen (e.g. from a portable concentrator), via common smooth bore oxygen tubing (this may require a reservoir to augment FiO2 and an adapter to connect to the device)
- high pressure (55psi/4bar) oxygen (from central pipes or a cylinder)
- high pressure air (usually not, as the turbine or compressor provides this)
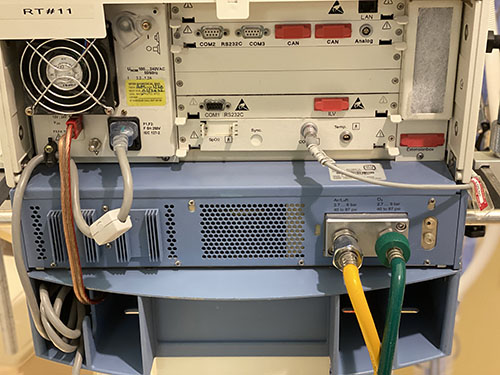
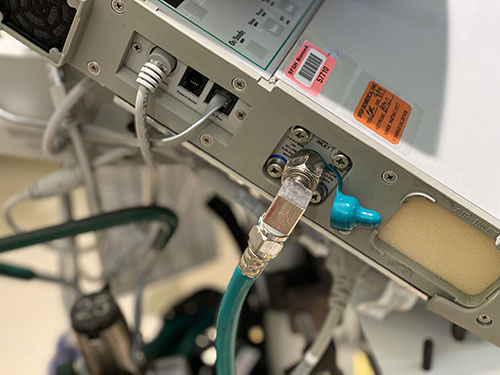
First recall the weight of atomic and molecular oxygen: O = 15.99 g/mol and O2 = 32 g/mol
Use the ideal gas law: PV = nRT
P = pressure (in atm); V = volume (in Liters); n = amount of substance (in moles); R = ideal gas constant = 0.0821 (units are (Liters*atm)/(Kelvin*n) ); T = absolute temperature (in Kelvin)
To get the gaseous volume of 1 mole of gas: V=nRT/P
(1 mol)(0.0821 L/atm/K/mol)(273K)/1atm = 22.4 L gas
1 mole gas (STP) = 22.4L = 32g of O2
(32g/mol)(1L/22.4L/mol)= 1.428 g O2 per gaseous liter at STP
Standard temperature and pressure is 1 atm and 273K or O C
If we assume a ‘room temperature’ of 22 degrees C, then:
V=nRT/P
(1 mol)(0.0821 L/atm/K/mol)(295K)/1atm = 24.2 L gas
(32g/mol)(1L/24.2 L/mol) = 1.322 g of O2 per gaseous liter at 22 degrees C
So if you have 1000 L of gaseous oxygen: 1.322 g/L (1000L) = 1.32 kg
The primary consideration should be to ensure that the oxygen system is dependable and sustainable. The best main supply source of oxygen to a patient is a direct piped system that connects to the patient bedside from a PSA plant (or oxygen reservoir supplied by a liquid oxygen source). The direct pipe system is highly recommended and preferred. The alternative is to bring cylinders to the bedside. The back-up supply source for a direct pipe system would be a manifold of cylinders and additional spare cylinders to adequately supply the pipe system if the main supply is temporarily not operating.
FAQ by Assist International
While this does occur, the short answer is ‘usually not.’
Atmospheric air (commonly referred to in clinical settings as ‘room air’) is composed of approximately 78.08% nitrogen, 20.95% oxygen, 0.93% argon, 0.04% carbon dioxide, variable amounts of water and negligible amounts of other gases. Oxygen concentrators use zeolite molecular sieves to separate oxygen from nitrogen in room air and produce a high concentration of oxygen. However, the sieve beds have a similar affinity for argon as they do for oxygen. This results in oxygen concentrators also performing as ‘argon concentrators,’ and the output of both gases increasing by nearly 5-fold in the output from a concentrator (~93-95% oxygen and 5% Argon).
There is no evidence of this concentration of argon (or much higher concentrations) having harmful effects in humans. There are data that argon, similar to some other noble gases (e.g. xenon) may have neuroprotective effects and at very high pressures (beyond seen in the clinical setting) may have some anesthetic properties (PMID: 31470983; PMID: 20472713; PMID: 19500647; PMID: 20236500).
High concentrations of argon will decrease the concentration of oxygen delivered to the patient, and this should be accounted for in the clinical setting. For most clinical settings and scenarios this is unlikely to be clinically significant. This is no different than many of the other gases commonly added to the breathing mixtures (e.g. nitrous oxide, helium, inhaled anesthetics).
The special case of low-flow, fully or partially closed circuit anesthesia is sometimes cited as a potential scenario where build of argon could occur at higher levels and cause potential harm. This has been studied and concluded that oxygen concentrators can be used for oxygen supply in fully or partially closed circuit anesthesia delivery systems for indefinite duration as long as the fresh gas flow (FGF) input flow rate is at least twice the oxygen consumption (e.g. FiO2 0.5 and FGF ~1 LPM), nitrous oxide is not used or is being accounted for, and the system is periodically opened for fully closed systems.
Unsurprisingly and unfortunately, arguments citing argon as a reason to not use oxygen concentrators in clinical care are usually driven by those with commercial interest in liquid oxygen.
References:
- Most portable oxygen concentrators use pressure swing adsorption to intake room air and output ~93-96% oxygen
- Most concentrators produce oxygen at 5 or 10LPM max, and at low pressure (this means far less than the 50psi/4bar needed to run HFNC, and most ventilators and NIPPV devices)
- There are a few portable devices capable of higher flow / high pressure output (e.g. 15-30LPM, or 50psi)
- Make sure the FiO2 is adequate (>~82-90%) at the max flow rate, as many concentrators make claims of high flows but deliver limited FiO2 (and thus limited benefit) at those flow rates.
- Performance of concentrators may vary by manufacturer in hot and humid environments (Peel et al, Anaesthesia, 2013)
- Zeolite does not last forever – needs to be replaced around 25,000 hours, though may be considerably less depending on use, maintenance and humidity
- While they can run continuously for long periods of time (months, years) they do require routine maintenance
- When run beyond max flow (flowmeter opened all the way), this can cause premature failure due to excess moisture building up in the sieve bed
Additional Resources:
- How to setup an oxygen concentrator by wikiHow
- Preventative maintenance for O2 concentrators – Med Equipment Troubleshooting – by Engineering World Health
- Frank’s Hospital Workshop Tutorials – Oxygen Concentrators – by Frank Weithoner
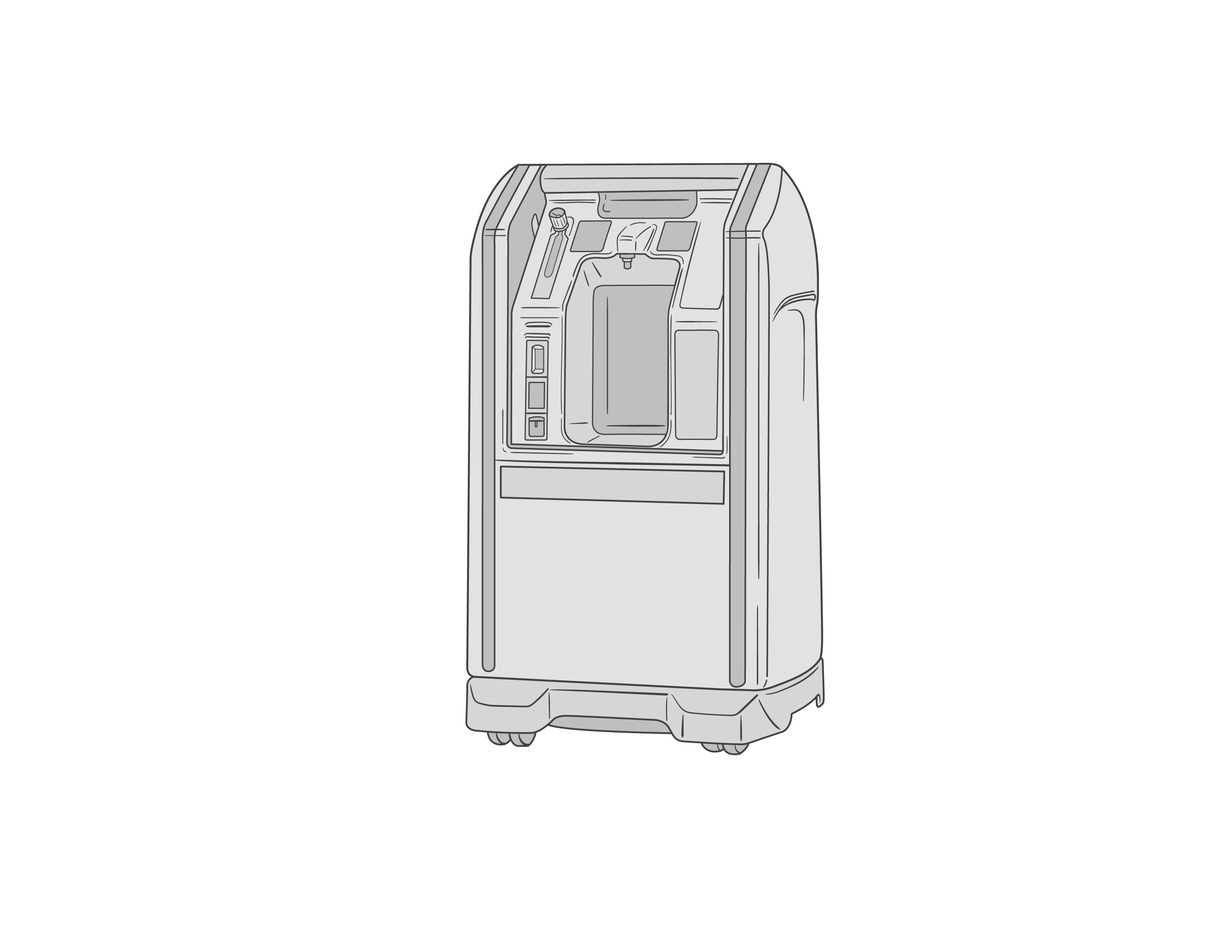
Database for oxygen concentrator specifications
- Supply shortages – Everyone in the world has been trying to buy these since the beginning of the pandemic. Be wary of large stockpiles of ‘available’ devices from unknown manufacturers.
- Flow rates – Concentrators commonly produce 3, 5, 8 or 10 LPM continuous flow output, though some may produce 10 or more LPM. All devices should have an adjustable flowmeter (and if planning to use for pediatric patients, a minimum flow rate of 0.5 LPM may be needed. Make sure the FiO2 is adequate (>~82-90%) at the max flow rate, as many concentrators make claims of high flows but deliver limited FiO2 (and thus limited benefit) at those flow rates.
- For stable patients on stable amounts of oxygen who are using the concentrators in climate controlled rooms (e.g. COPD at home, one of the original use scenarios for portable concentrators), a device with relatively low output < 5LPM may be sufficient
- For hospitalized patients who may have fluctuating needs (e.g. acute respiratory illness, COVID19), concentrators with higher outputs (e.g. 10-15 LPM, or higher) may be more desirable
- Oxygen concentration – WHO Technical Specifications stipulate output of >82% concentration of oxygen at max rated flow rate at 40 degrees C and 95% relative humidity (RH). Most high quality devices should have >90% output. NOTE: many concentrators, especially smaller (<10kg) units only produce 30% FiO2 at max flow rates, which is inadequate to care for most patients with acute respiratory illnesses.
- Power – ensure compatibility with local power supply and outlet adapters; and ensure efficiency <70W/LPM. Many oxygen concentrators do not have backup batteries that will power the device in the event of power failure. Consider using with a voltage stabilizer and surge protector.
- Noise – <50 dBA; many noisy units on a single ward can make it difficult to hear patient alarms
- Durability – Consider IP21 or greater to offer special protection against moisture
- Outlet pressure – must generate at least 55kPa at all flows and ensure stable (narrow range) of output pressure (+/- 1 psi)
- Operating environment – ensure safe operation in 0 to 40 degree C, and RH 15 to 95%; ensure certified to altitude suitable for destination (many are not certified above 3000m due to partial pressure changes)
- Alarms – device must have alarms for low oxygen concentration alarm (<82%), no flow, high/low pressure, low battery, power supply failure, high temperature. NOTE: be cautious of devices with prolonged warm up periods, maximum operating durations (good units should work continuously), or auto-shutoff functions.
- Spare parts – ensure spare parts for one year – including spare battery for power failure alarms (more recent models may use a capacitor and do not have a battery), intake filter, and internal filter
- Delivery devices – don’t forget to ensure access to delivery devices (e.g. nasal cannula, face masks and humidification bottles)
- Access to maintenance tools – ideally facilities should have pressure gauge, FiO2 meter oxygen analyzer, and spare parts for routine maintenance; consider a local service contract or identifying a provider
- Training – recipients of concentrators should be provided with training on operation, troubleshooting and maintenance
- Be wary of devices with auto-shutoff timers as these can increase likelihood for errors
Additional Resources:
- Evaluation of O2 concentrators for use in countries with limited resources
- How to setup an oxygen concentrator by wikiHow
- Preventative maintenance for O2 concentrators – Med Equipment Troubleshooting – by Engineering World Health
- Frank’s Hospital Workshop Tutorials – Oxygen Concentrators – by Frank Weithoner
- WHO Technical Specifications for oxygen concentrators
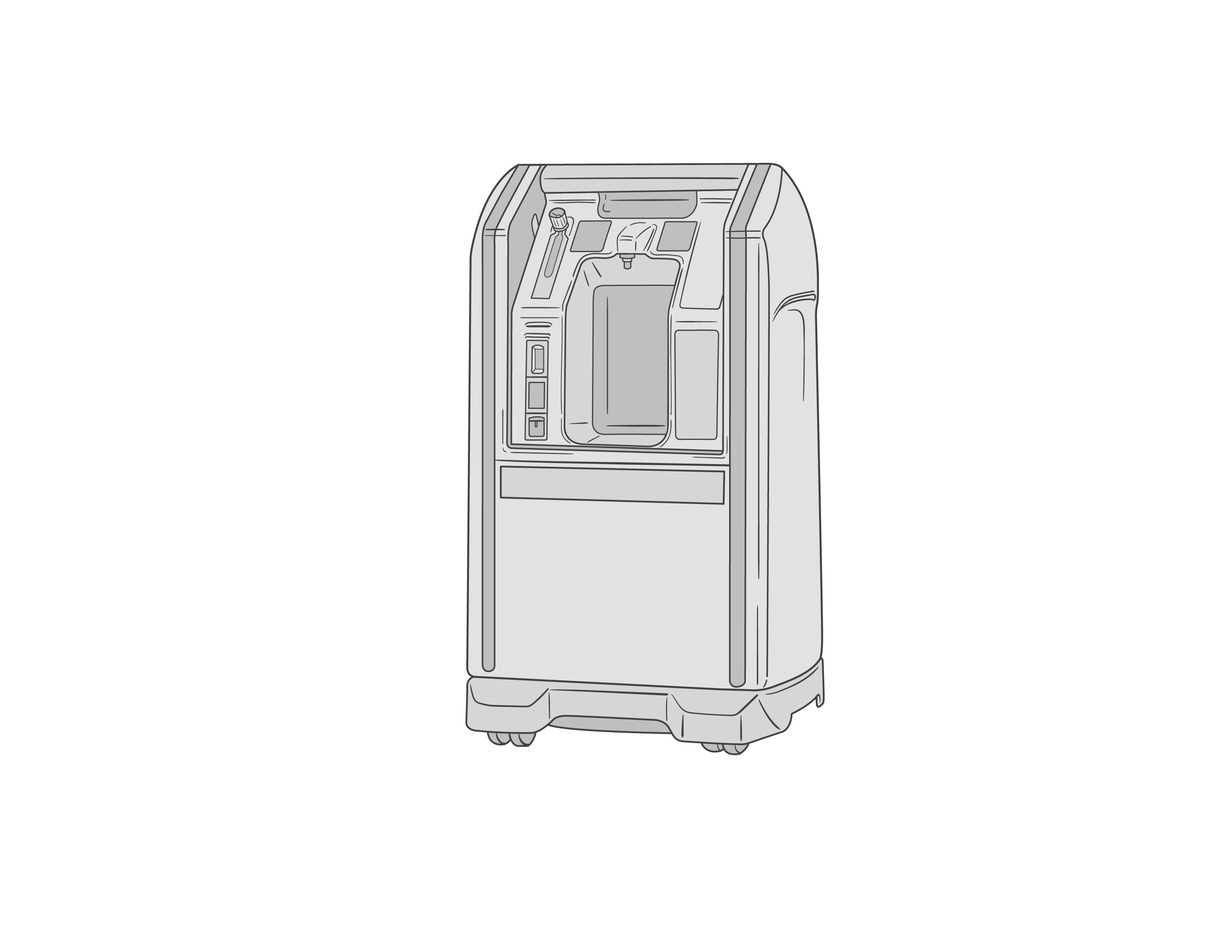
High pressure oxygen sources are capable of delivering oxygen at ~50psi/4bar to a device. These include oxygen cylinders (via regulator), oxygen plants via a compressor, liquid oxygen (via vacuum insulated evaporators) and very few portable oxygen concentrators (via additional compressor). High pressure oxygen is required for most ventilators, high flow nasal cannula and non-invasive positive pressure ventilators when taking care of critically ill patients.
Low pressure oxygen sources deliver oxygen at far less than <50psi/4bar. These include oxygen from a low flow flowmeter or a portable oxygen concentrator. Generally, these cannot deliver high enough oxygen concentrations to take care of severely hypoxemic patients.
***Some ventilators (e.g. LTV2200, Zoll 731) can operate with either high pressure or low pressure oxygen input. Other ventilators (e.g. PB560) may only be capable of utilizing a low pressure oxygen input. Of note – when utilizing low pressure oxygen input, some ventilators may not be able to provide high enough concentrations of oxygen to care for critically ill patients. Check manufacturers’ reports for maximum oxygen concentration delivery.
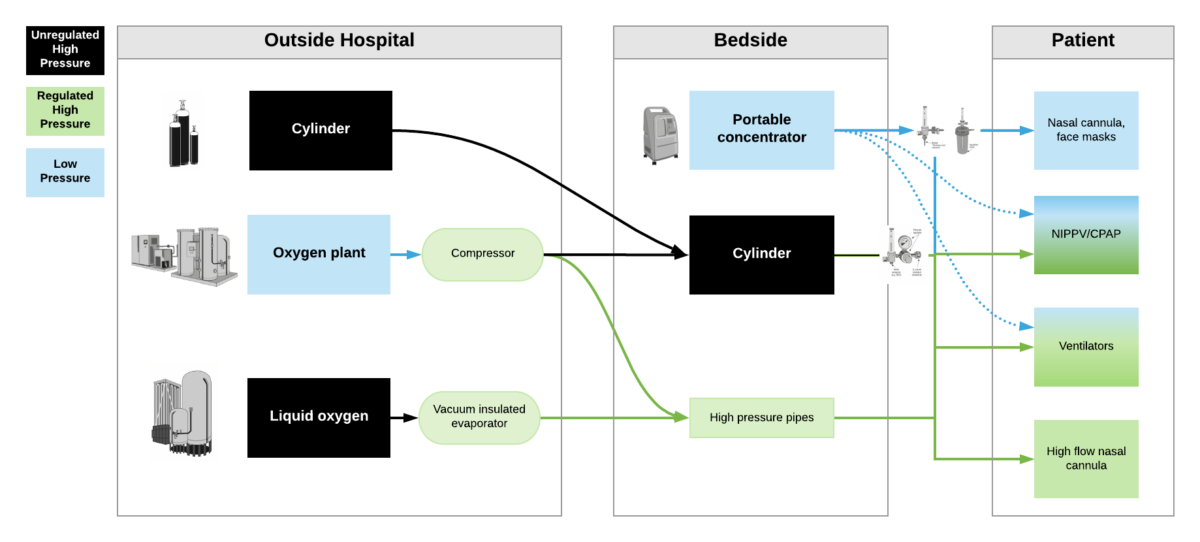
- The short answer is that it depends on many factors and varies by ventilator model (so check the manufacturers’ specifications)
- Some ventilators like the LTV 1200/2200, PB560 and Zoll 731 can run off of a low pressure oxygen supply. They utilize 21% ambient air from the room via a built in compressor or turbine and mix that with the low pressure oxygen input.
- When utilizing low flow oxygen, ventilators usually will not allow you to use the blender – i.e. you can’t set the FiO2
- Concentration of oxygen delivered to the patient (FiO2) is determined by: minute ventilation, flow of oxygen supply into the vent, concentration of oxygen supply into the vent, and potentially other factors.
- For example:
- LTV 1200/2200 – If input flow is set to minute ventilation, then the LTV1200/2200 can deliver ~100% FiO2 up to ~20LPM minute ventilation. For a patient with 10 LPM minute ventilation and 5 or 10LPM input low pressure oxygen, max FiO2 delivered to the patient is approximately 50 and 75% respectively. (See Figure below left)
- Zoll 731 – If input flow is set to minute ventilation, and an external reservoir (e.g. ZOLL 731) is used on the compressor intake, then FiO2 of ~100% is possible on the Zoll 731 for minute ventilation up to ~20 LPM. (See Figure below, bottom) For more information on using the ZOLL 731 with low pressure O2 sources see the users manual and this quick reference.
- PB560 – Some ventilators like the PB560 that only accept low flow oxygen may be limited in the amount that can be delivered into the device at low pressure and thus be limited in FiO2 delivery to the patient (e.g. the PB 560 is designed to deliver a percentage of oxygen equal or lower than 50%. Exceeding this value may cause the ventilator to malfunction and put the patient at risk). (See Figure below right)
- Of note, 50% FiO2 is approximately equivalent to that delivered by a simple facemask at 10LPM (Read more about FiO2 delivered by various oxygen delivery devices)
- When using low pressure oxygen supply for devices with bias flow (flow during the expiratory time) and/or during NIV modes with leak compensation, the FiO2 can be diluted significantly.
- When using low pressure oxygen supply, you likely will need to change the ventilator setting to indicate such a source is being used (e.g. ‘reservoir mode’ or ‘low pressure input’)
- Due to slight difference in the densities of air and O2, tidal volume may decrease slightly as FiO2 increases via low pressure entrainment. See manufacturer’s specifications, though generally this would be expected to be less than 10% change in tidal volumes.
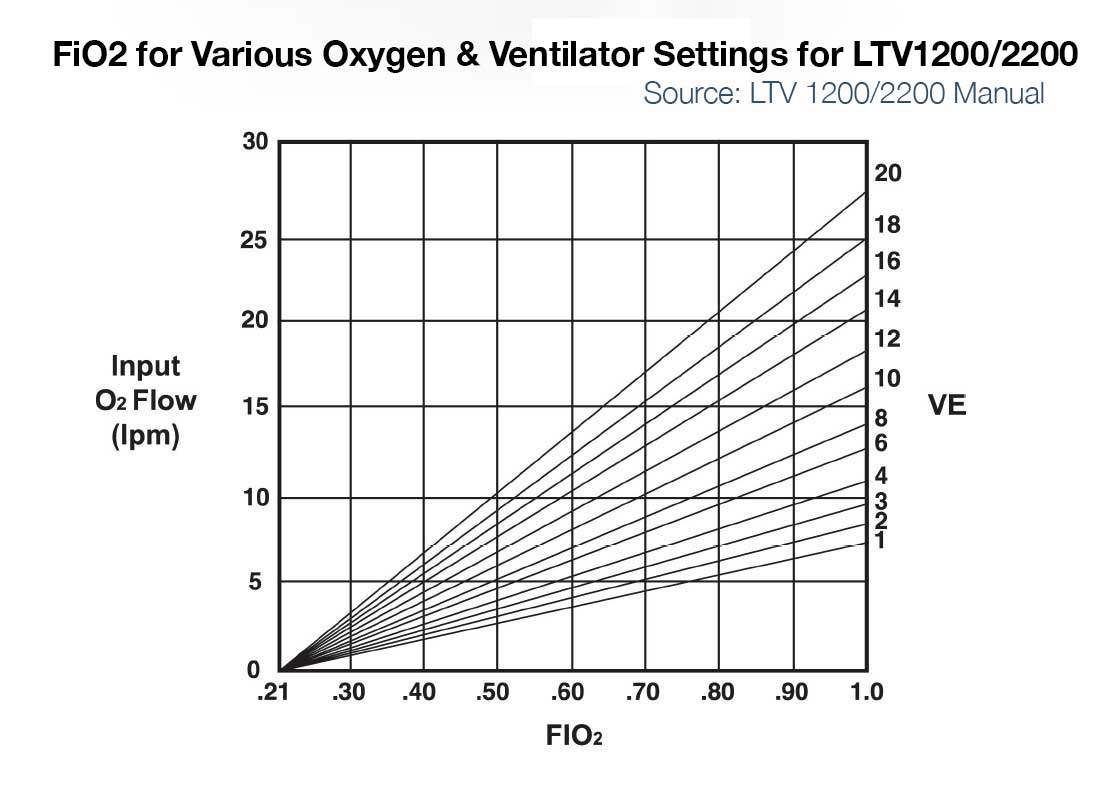
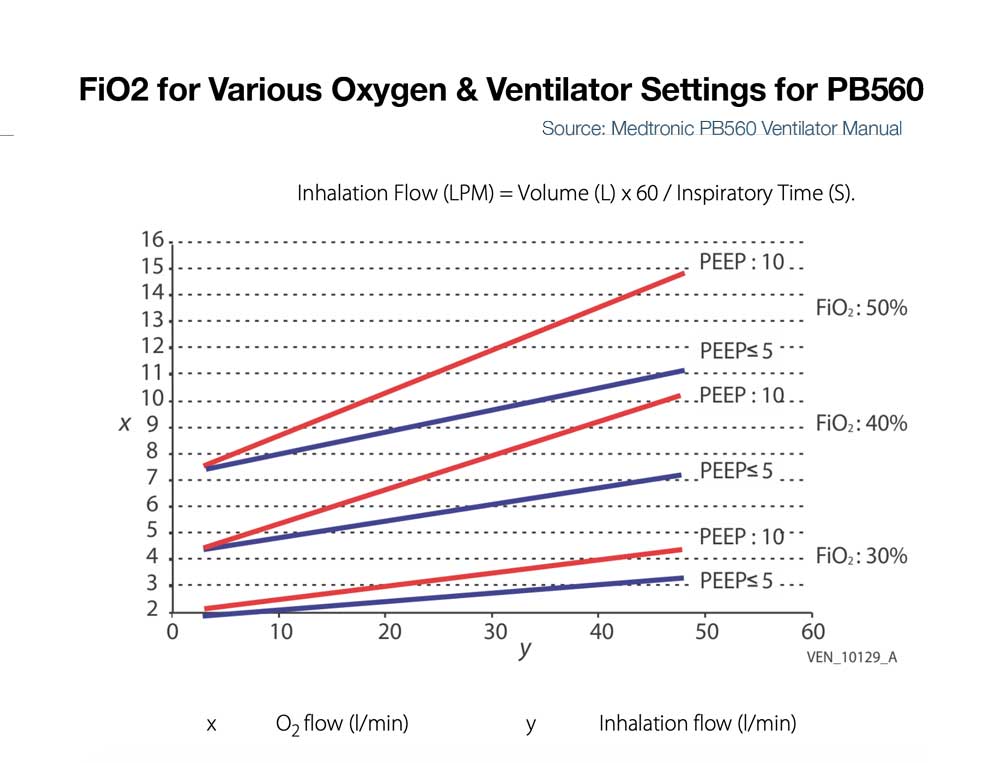
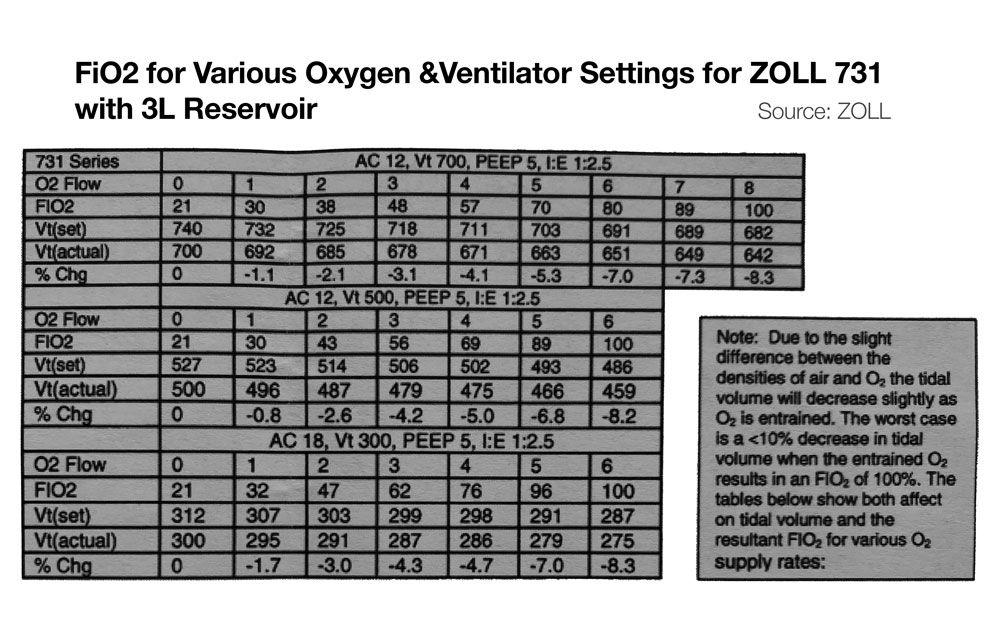