Oxygen FAQ
Up to date, expert answers to frequently asked questions (FAQ) about oxygen supply systems, respiratory care and pulse oximetry written by OCC & collaborators.
How pulse oximeters work
Some oximeters, sometimes. Blood pressure fluctuations occur with respiratory (or ventilator) cycles, and changes in the arterial waveform signal due to pulse pressure variation can often be more pronounced in hypovolemic patients. For this reason, pulse pressure variation (PPV) or pulse oximetry plethysmographic signal (POP) are sometimes used to try to assess volume responsiveness in patients.
Similar to a peripheral arterial pressure waveform, many pulse oximeters show a processed representation of the photoplethysmographic waveform. However, visual estimation of variations in the pulse oximeter waveform are unreliable. Some pulse oximeters will populate PPV or POP values that are automatically and continuously calculated based off of the waveform. However, each pulse oximeter manufacturer uses different algorithms to calculate these values and displays, making it very difficult to interpret accurately and clinically.
Some studies have stated that POP appears to be effective for predicting fluid responsiveness in ventilated patients, while others have found that it did not correlate well with arterial pressure waveforms. Overall, using pulse oximeters in isolation to evaluate volume responsiveness is not adequately validated or reliable in the clinical setting at this time. This is due to many limitations of this technique including poor or noisy signal, probe position, motion, vasoconstriction, and highly processed pleth signals which vary among manufacturers.
References: Hess, Respir Care 2016; Sandroni et al, Intensive Care Med 2012; Addison, Anesth Analg 2014; Monnet et al, Crit Care 2005; Antonsen et al, Anesthesiol Res Pract 2012
Keywords: pulse pressure variation, PPV, volume, fluid, POP
Pulse oximeters estimate the SpO2 of arterial blood, isolated from other tissues (including venous blood), by applying the Beer-Lambert Law as arterial blood volume fluctuates throughout the cardiac cycle. The Beer-Lambert Law of absorbance demonstrates that the transmission of light is attenuated as it passes through different optical filters (tissue types). Oxygenated hemoglobin and deoxygenated hemoglobin have unique molar extinction coefficients for different wavelengths of light. As arterial blood volume increases during systole the amount of oxygenated hemoglobin increases and ratio of red to infrared light changes (known as the modulation ratio).
Conversely, the blood volume and therefore the amount of oxygenated hemoglobin changes very little throughout the cardiac cycle in other tissue types. By taking the amount of change in absorbance over the change in time (pulse rate), the non-arterial tissues are effectively eliminated mathematically (see below) and the amount of oxygenated hemoglobin in arterial blood remains.
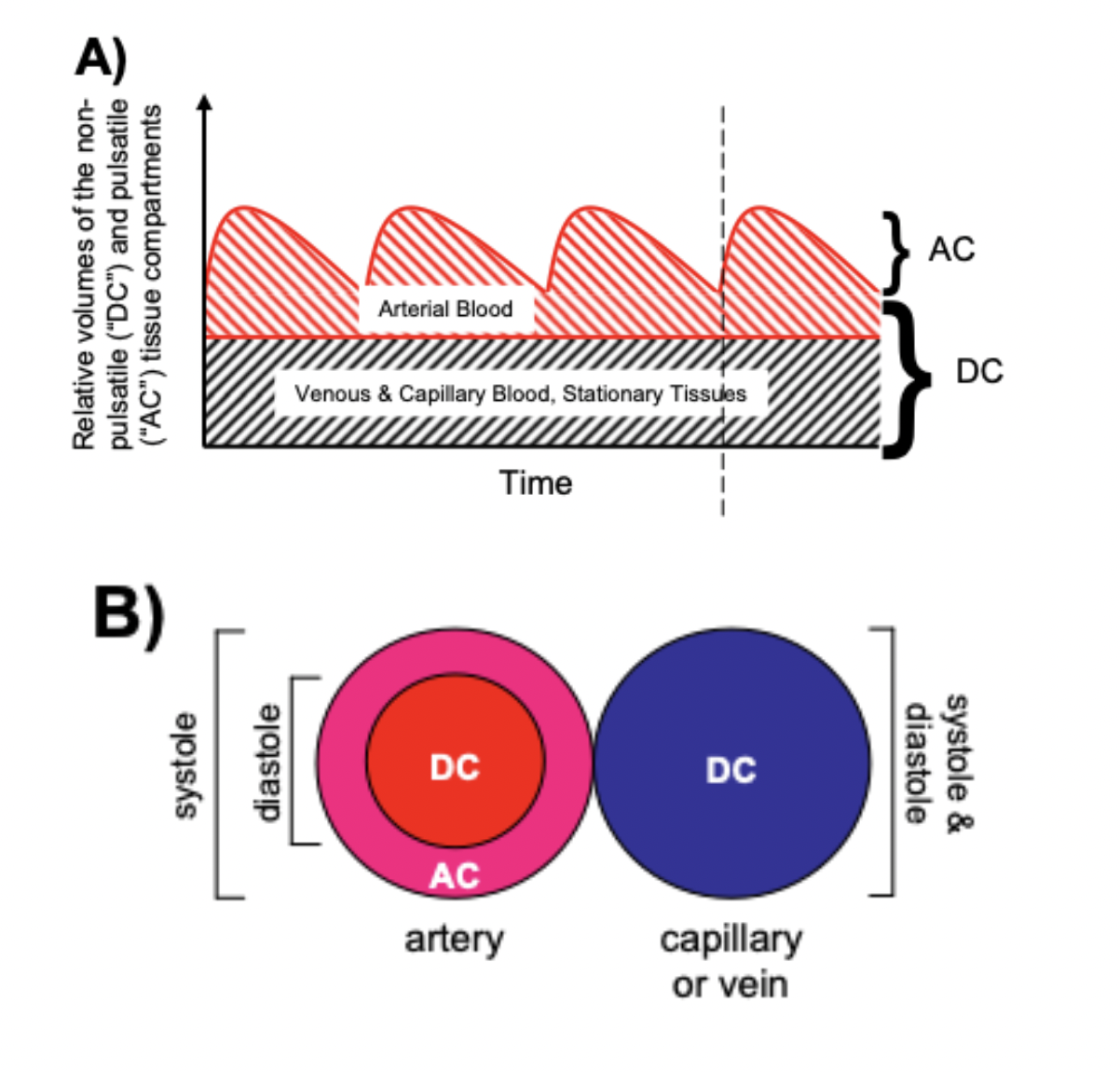
Mathematically (for transmittance pulse oximeters):
Light from the light-emitting diode is absorbed as it passes through various tissue types before being measured by the photodiode detector.
Absorbance(total) = sum(Absorbance(all tissue types)) Formula 1
Absorbance(total) = Absorbance(arterial blood) + Absorbance(venous blood) + Absorbance(skin, varies by pigment type or melanin content) + Absorbance( other tissue types fat, bone, vessel walls, etc) Formula 1a
Taking the derivative, or measuring the change in Absorbance(total) over the change in time gives the following:
dAbsorbance(total)/dt = dsum(Absorbance(all tissue types))/dt Formula 2
dAbsorbance(total)/dt = dAbsorbance(arterial blood)/dt + dAbsorbance(venous blood)/dt + dAbsorbance(skin)/dt + dAbsorbance(other tissue types)/dt Formula 2a
As dAbsorbance for non arterial tissue types is essentially zero throughout the cardiac cycle, dAbsorbance(total)/dt reduces to:
dAbsorbance(total)/dt = dAbsorbance(arterial blood)/dt Formula 3
Absorbance = the negative log of transmission, and Formula 3 can be written as:
R = [dlog(transmission)/dt]wavelength1/[dlog(transmission)/dt]wavelength2 Formula 4
(R = Modulation Ratio, wavelength 1 and 2 are wavelengths of red light and infrared light)
or alternatively, as:
R = (AC/DC)wavelength1/(AC/DC)wavelength2 Formula 5
(AC = alternating component and DC = constant(direct) component)
Without an adequate pulse, pulse oximeters will either be unable to measure SpO2 or will report inaccurate SpO2 levels.
References: Chan et al, Respiratory Medicine 2013 Mannheimer Anesth Analg. 2007
Keywords: modulation, ratio
A transmittance pulse oximeter contains two small light-emitting diodes (LED) which each emit a specific wavelength of light, 660 nm (red) and 940 nm (near infrared). Oxygenated Hemoglobin (O2Hb), absorbs a greater amount of 940 nm wavelength light and a lower amount of 660 nm wavelength light than does deoxygenated hemoglobin (HHb). Opposite from the LED, a transmittance pulse oximeter utilizes a photodiode to measure the amount of 940 nm and 660 nm wavelength light that is transmitted through the body part between the LED and photodiode. A microprocessor then calculates SpO2 using the ratio of the measured absorbances over a series of pulses based on individual device calibration to determine the modulation ratio and its corresponding SpO2.
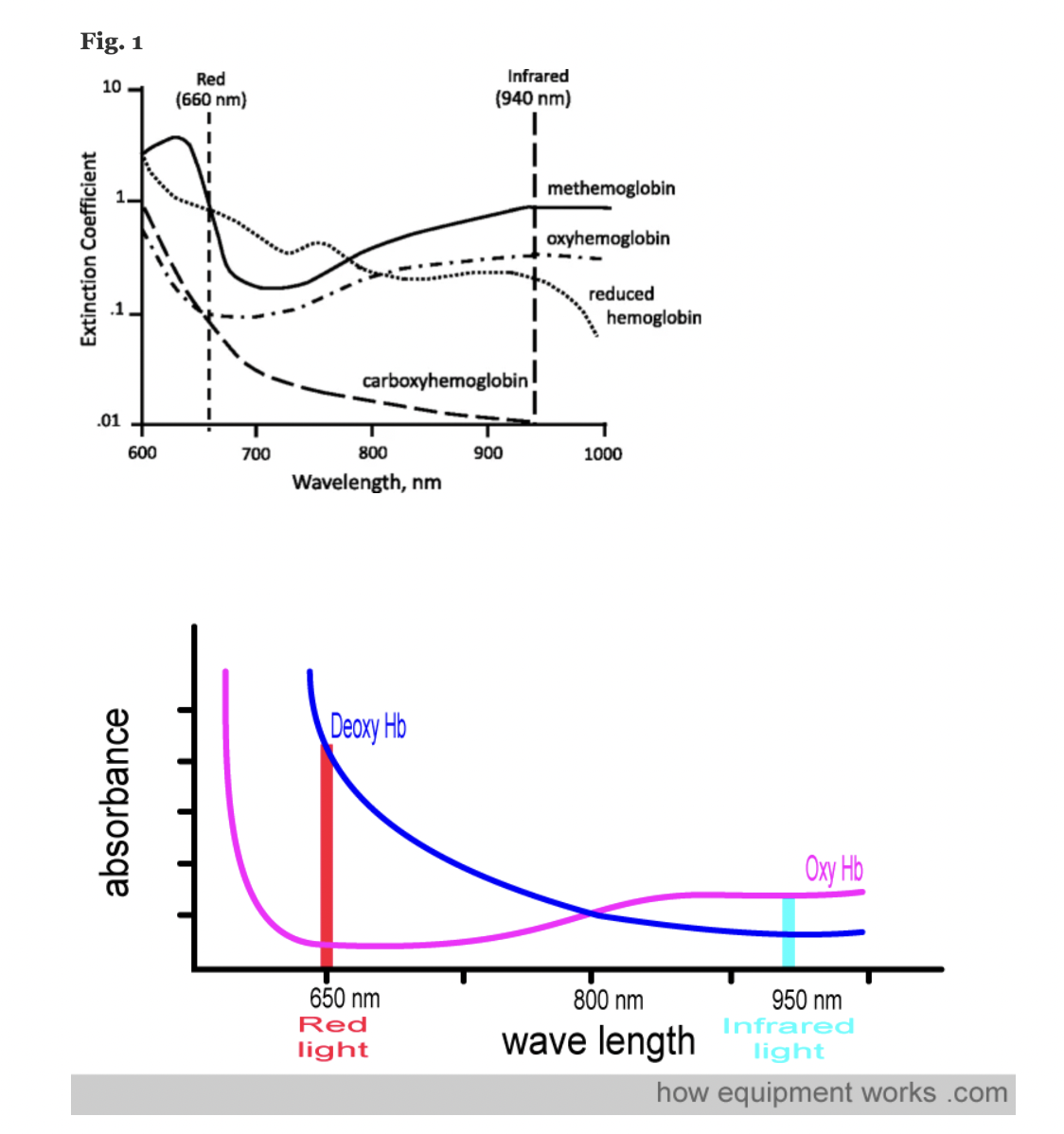
Figure: Jubran, Critical Care, 2015
References: Chan et al, Respiratory Medicine 2013 Lifebox Pulse Oximetry Learning Module; WHO Using Pulse Oximeters Jubran, Critical Care, 2015 How Equipment Works – Pulse Oximeter
Keywords: wavelengths, IR, infrared, red, LED, photodiode, hemoglobin
A pulse oximeter is a noninvasive monitoring device that can indirectly measure a person’s functional oxygen saturation (SpO2) and help with early detection of hypoxemia. Pulse oximeters work by either transmitting light through tissue perfused by blood (ideally a site with a dense capillary bed like the fingertip). They are called pulse oximeters because they use the pulsations to discriminate between arterial blood and other tissue or venous blood. The probe on the pulse oximeter has light-emitting diodes (LEDs) which shine at least two types of light, red and infrared. The device is positioned so that the light shines through a translucent part of a patient’s body, such as a fingertip or earlobe, and measures the changing absorbance at each wavelength. Oxygenated hemoglobin absorbs more infrared light, whereas deoxygenated hemoglobin absorbs more red light. Since the absorption of light at these wavelengths differs between oxygenated and deoxygenated blood, the device can determine the absorbances due to just the arterial blood and thereby determine a patient’s peripheral oxygen saturation.
References: Lifebox Pulse Oximetry Learning Module; WHO Using Pulse Oximeters
Keywords: wavelengths, IR, infrared, red, LED
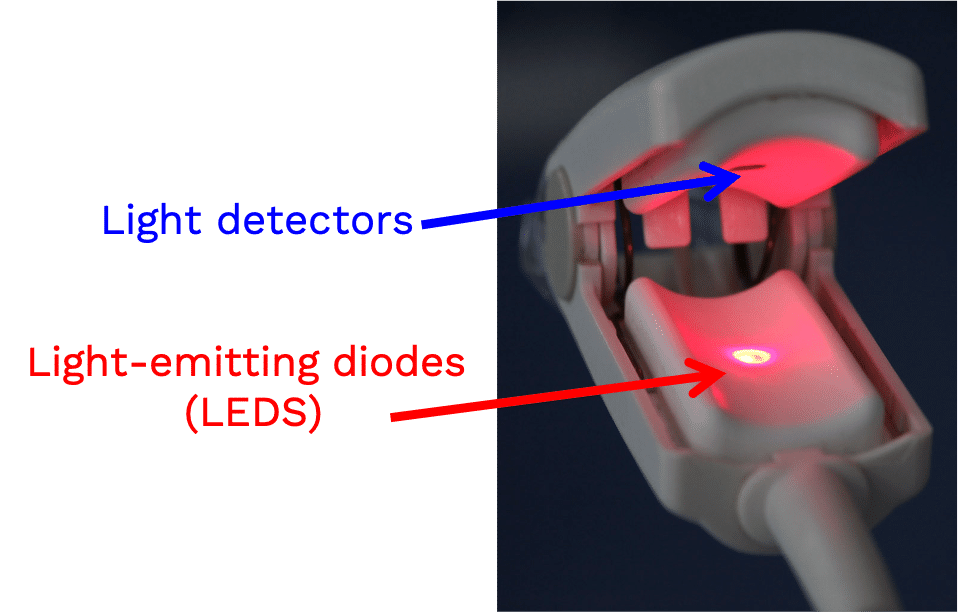
A pulse oximeter photoplethysmograph (commonly referred to as a ‘pleth’) is a graphical display of the pulse oximeter signal over time. Its appearance can vary widely under different clinical scenarios. In healthy patients, the graph should appear as asymmetric humps similar in appearance to an arterial pressure waveform though usually with less level of detail (i.e. the dicrotic notch may not be visible). The waveform should appear at intervals that match the heart rate and regularity.
Patient motion, tremor and poor perfusion are common factors that affect the pleth. The pleth is an extremely useful feature to help clinicians quickly determine the quality of the oximeter signal. Of note, in the face of a low signal (or low perfusion) some oximeters may auto adjust the scale of the display (y axis) to increase the visual amplitude of the pleth. This can be misleading to clinicians who must also pay attention to signal quality indicators when available.
References: Jubran, Crit Care 2015
Keywords: pleth, waveform, PPG
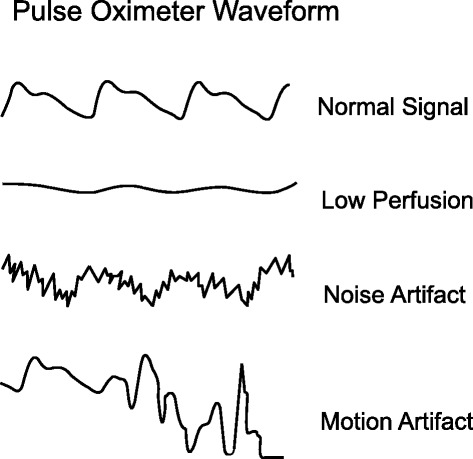
A molar extinction coefficient is a measure of the absorption of light at a particular wavelength by a substance of known concentration. Specifically, it is the measure of how much light is absorbed per unit length (i.e. centimeters) by one mole of the substance.
In pulse oximetry, the molar extinction coefficient is used to calculate the concentration of oxygenated and deoxygenated hemoglobin in blood based on the absorption of light at two different wavelengths (usually 660 nm and 940 nm) by the hemoglobin molecules.
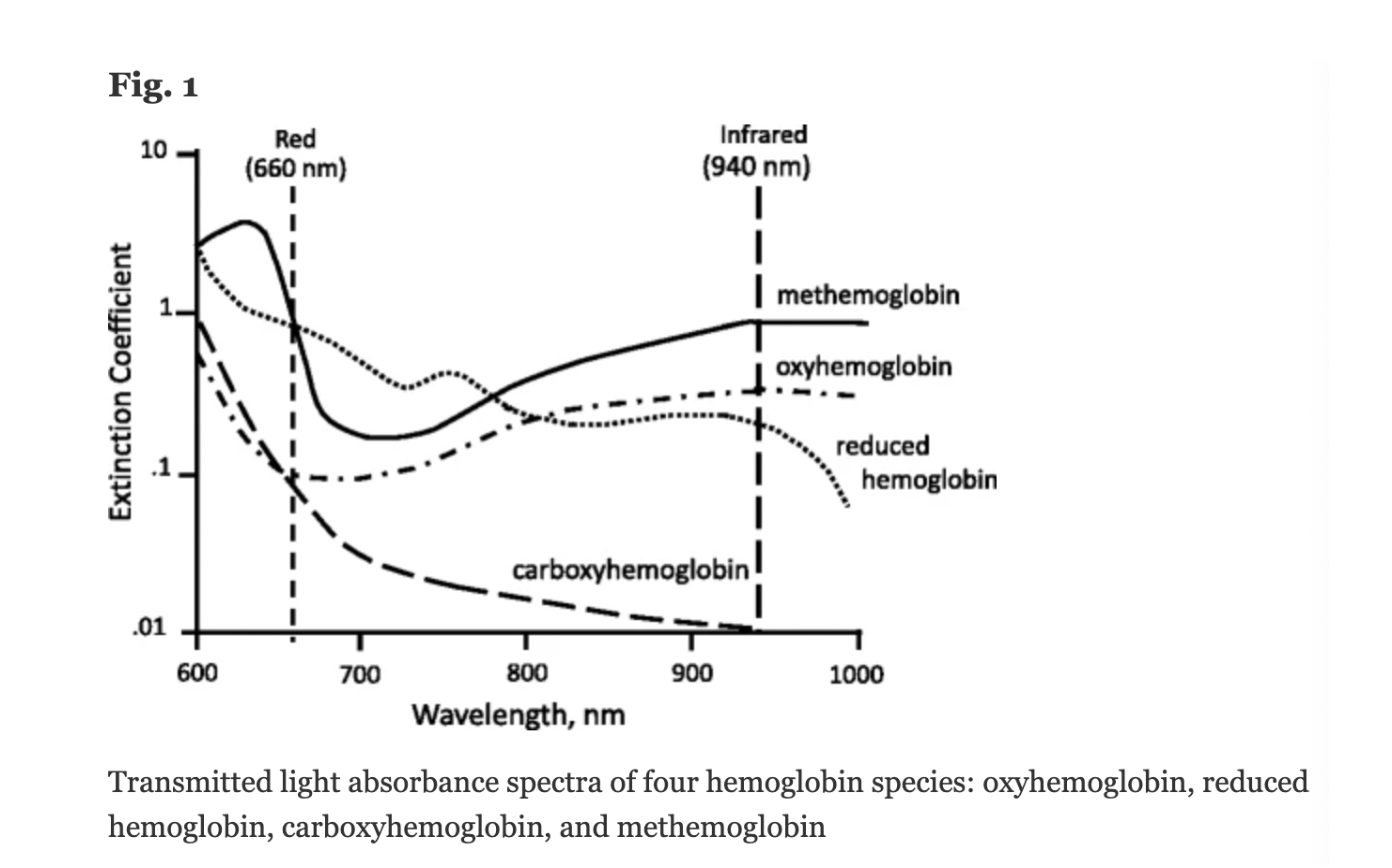
Figure: Jubran, Critical Care, 2015
Keywords: molar extinction coefficient, extinction coefficient, hemoglobin,
References: Jubran, Critical Care, 2015 Chan et al, Respiratory Medicine 2013
The term CO-oximetry refers to devices that use at least four wavelengths of light to measure not only oxy and deoxy-hemoglobin, but also other forms of hemoglobin (e.g. CO-Hb, Met-Hb). While most conventional pulse oximeters use two wavelengths of light and can only detect oxyhemoglobin or deoxy-hemoglobin (to provide a functional saturation, sO2), some pulse oximeters contain many more wavelengths and the ability to function as ‘pulse CO-oximeters.’ With more wavelengths, these devices can measure dyshemoglobins and provide “fractional” oxygen saturation – see image below. (Note: these devices are clearly marked with this function and often are considerably more expensive than other devices).
Despite this, the term ‘CO-oximeter’ is often used synonymously with ‘arterial blood gas analyzer’ because historically it was only multi-function arterial blood gas analyzers that possessed CO-oximetry functionality. Some pulse CO-oximeters have ~10 wavelengths of light, whereas benchtop CO-oximeters may use 128 to 256 wavelengths of light.
Of note, the terms “CO-oximeter” and “Hemoxemeter” are brand names coined by the companies Instrumentation Laboratories and Radiometer, respectively. The generic term for a device with these capabilities is a “multi-wavelength oximeter,” but the term “CO-oximeter” is widely used to refer to these devices, regardless of brand.
Keywords: CO-oximetry, arterial, carboxyhemoglobin, methemoglobin
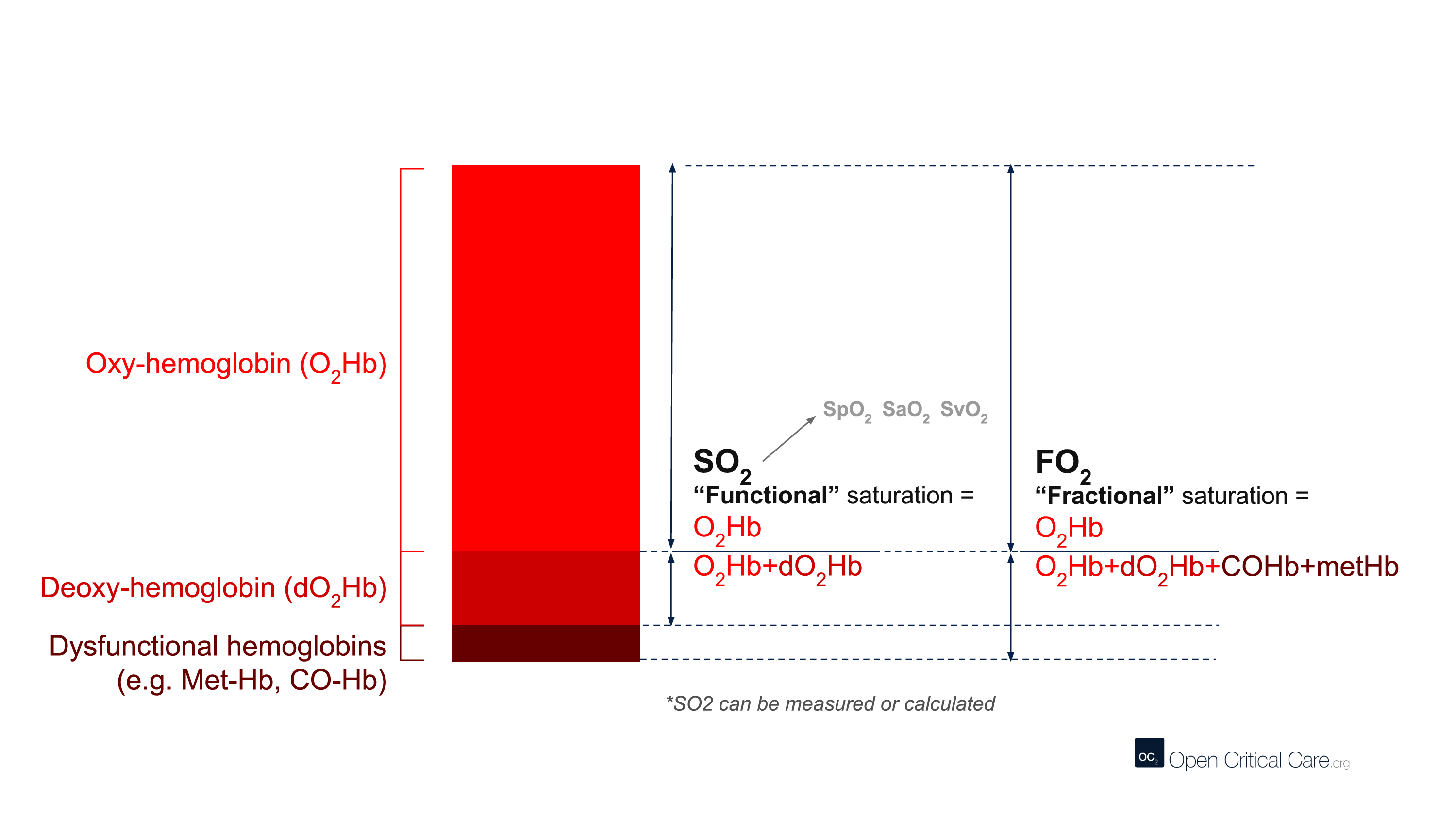
Spectrophotometry is a type of quantitative measurement technique that is used to measure the reflection or transmission properties of a substance as a function of wavelength. Every type of substance absorbs light over a specific range of wavelengths. This type of measurement allows us to assess the intensity of light that a substance (such as hemoglobin in blood) absorbs, and therefore has clinical and bioengineering applications.
Clinically, spectrophotometry is used in pulse oximeters to determine the proportion of oxygenated hemoglobin in arterial blood. Since different wavelengths of light are absorbed by oxygenated and deoxygenated blood, pulse oximeters can use this technique to determine a patient’s peripheral oxygen saturation (SpO2).
Keywords: spectroscopy, spectrophotometry, wavelength, light
There are two main types of pulse oximeter: transmittance devices and reflectance devices. Transmittance pulse oximeters are the most common and work by transmission (i.e. shining a light) through tissue, usually a fingertip or ear. As the light passes through the body part, the amount of oxygen in the blood determines how much light is absorbed in the tissue. A light detector on the other side of the probe detects light that is not absorbed, and a microprocessor calculates the oxygen saturation in the blood. Reflectance pulse oximeters are placed on the skin surface and measure the light reflected off the tissues rather than through the tissue. In this way, absorption is measured to calculate oxygen saturation. Of note, reflectance devices are inherently more difficult to design to perform well.
References: Lifebox Pulse Oximetry Learning Module
Keywords: transmittance, reflectance, light, absorption
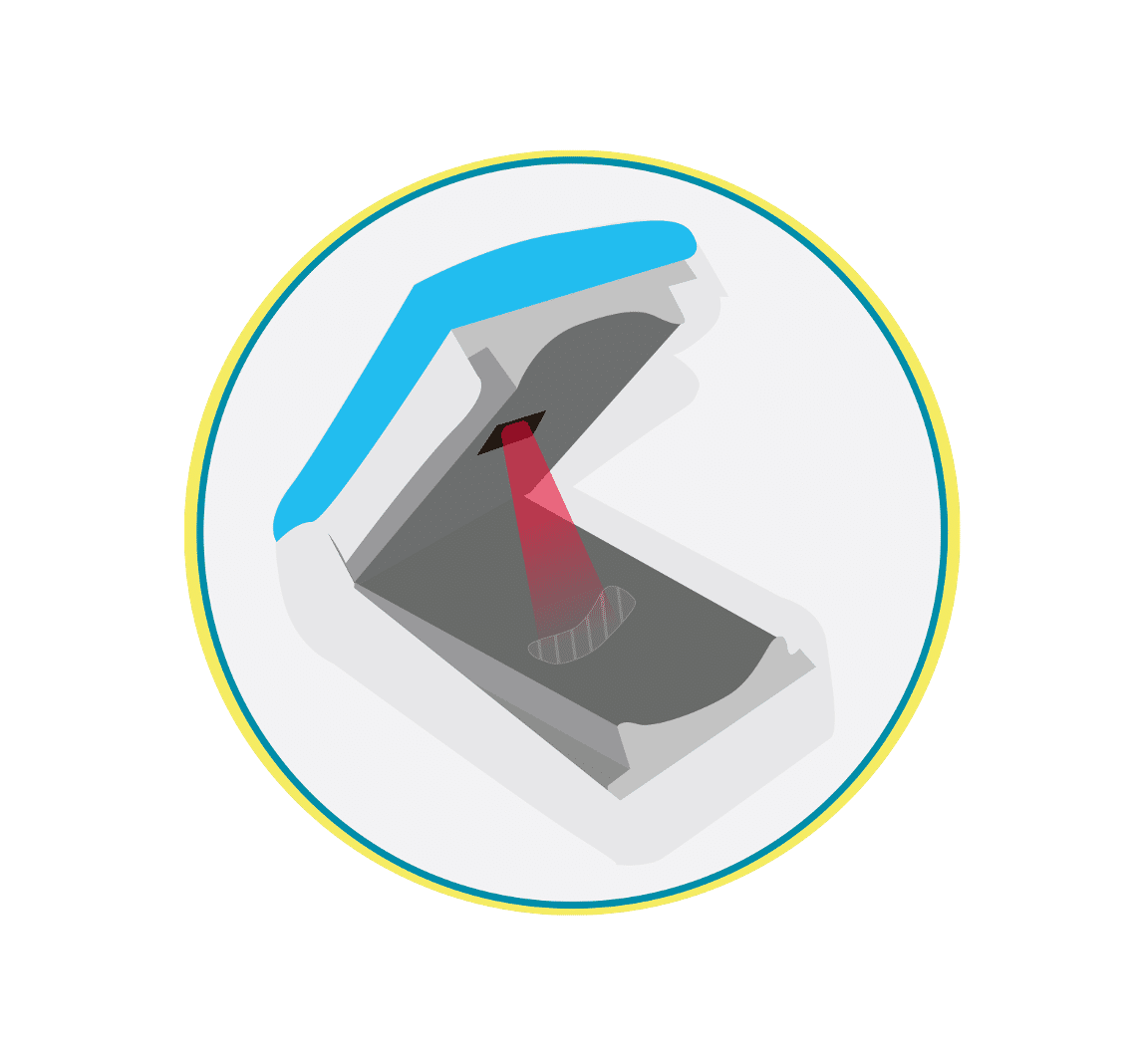
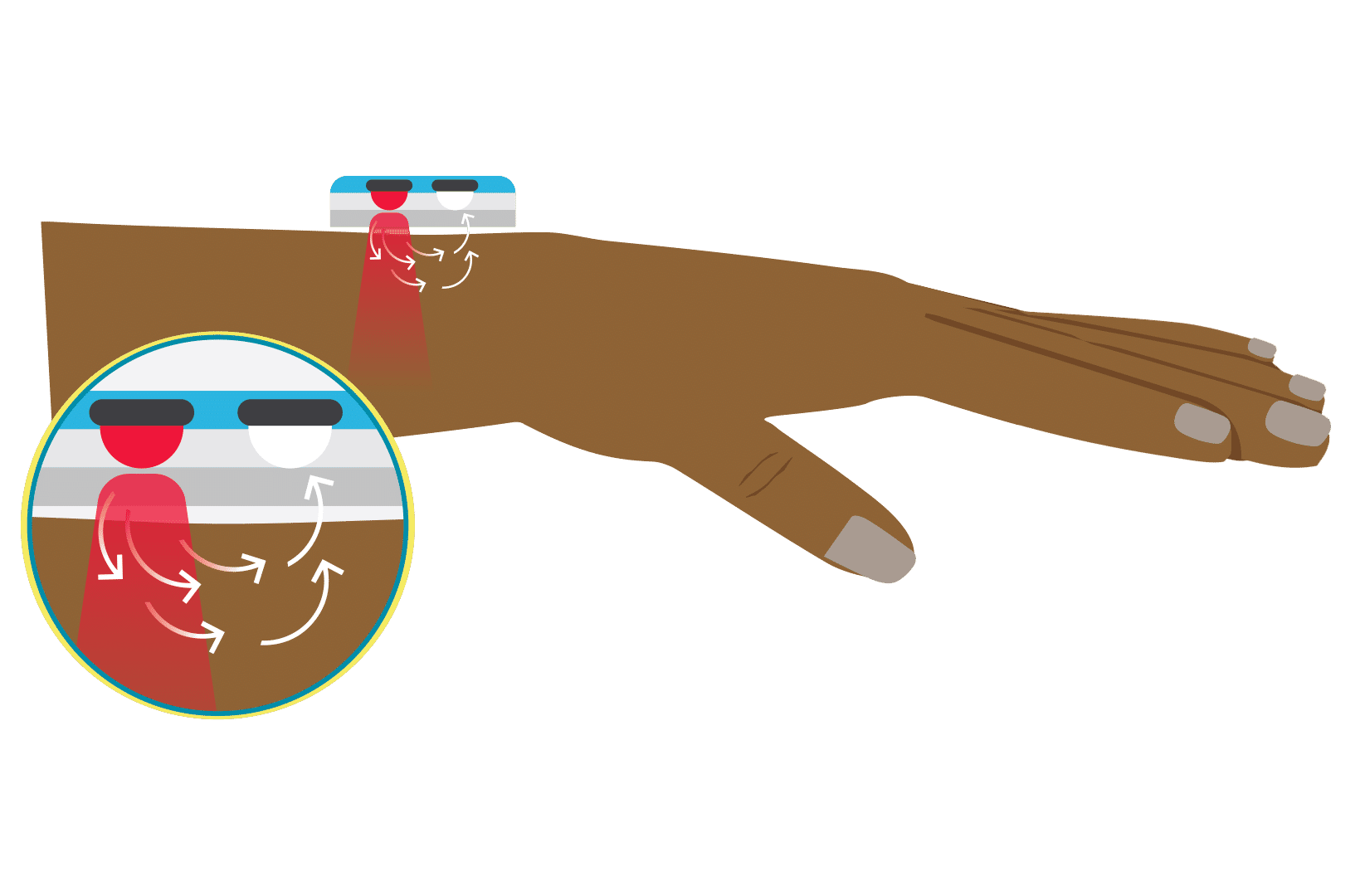
In the context of pulse oximetry, the modulation ratio is a measure of the amplitude modulation of the light transmitted through the tissue due to the presence of pulsatile arterial blood. It is defined as the ratio of the AC (alternating current) component to the DC (direct current) component of the transmitted light signal
The modulation ratio is used in pulse oximetry to determine the accuracy and reliability of the measured oxygen saturation (SpO2) values. A higher modulation ratio indicates a stronger pulsatile signal and better signal-to-noise ratio, resulting in more accurate and reliable SpO2 readings.
According to a study by Allen et al. (2007), the modulation ratio is typically in the range of 1% to 3% for healthy adults. However, the modulation ratio may be lower in patients with low perfusion, weak pulse, or other physiological conditions that affect the pulsatility of arterial blood flow. In such cases, the accuracy and reliability of the SpO2 readings may be compromised.
Keywords: modulation ratio, R-value
Reference: Allen, J. (2007). Photoplethysmography and its application in clinical physiological measurement. Physiological Measurement, 28(3), R1-R39.
In 1940, J.R. Squire was the first to recognize that the differences in transmission of red and infrared light permitted oxygen saturation to be computed. In 1942, the British scientist Glen Millikan developed the first portable pulse oximeter to be used during pilot training in World War II. The device was placed on the earlobe and was used to monitor pilots’ oxygen saturation and safety during flight.
Thirty years later in 1972, the Japanese bioengineer Takuo Aoyagi developed conventional pulse oximetry by using the ratio of red to infrared light absorption in pulsating (arterial) blood to calculate oxygen saturation. This was later commercialized and gradually integrated into clinical practice in the 1980s and 1990s. Notably, the American Society of Anesthesiologists determined in 1986 that using pulse oximetry was to be recognized as the new standard of care. To learn more history about the development of the pulse oximeter, read here.
References: Severinghaus et al, J Clin Monit 1986; Millikan, Rev Sci Instrum 1942; Severinghaus, Anesth Analg 2007, Wood Library Museum of Anesthesiology
Keywords: first, invention, Millikan, Aoyagi
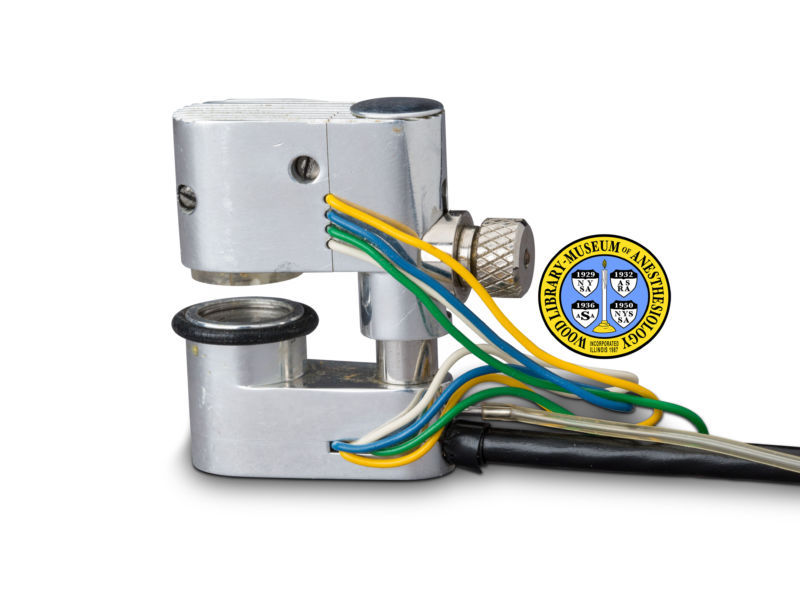
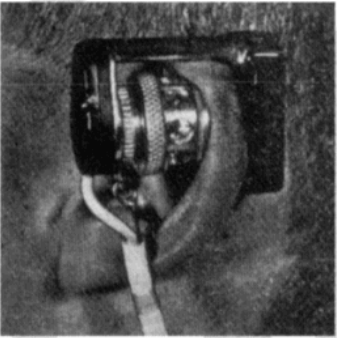
Conventional pulse oximeters assume that the major absorbers of light within arterial blood are deoxygenated hemoglobin (HHb) and oxygenated hemoglobin (O2Hb) and calculate SpO2 based on the ratio of measured absorbance of only two wavelengths of light (660nm and 940nm). Methemoglobinemia (MetHb) occurs when the iron in hemoglobin is oxidized, altering its ability to bind and offload oxygen.
The molar extinction coefficient of MetHb at 660 nm red light is approximately 1 and approximately equal to the molar extinction coefficient of HHb (see image below). The molar extinction coefficient of MetHb at 940 nm infrared light is also approximately 1 and significantly higher than the molar extinction coefficient of O2Hb. As a result of MetHb absorbing 660 nm and 940 nm light approximately equally, the Modulation Ratio (R-Value) approaches 1, this corresponds to an SpO2 of 80-88%. This can result in either an overestimation or an underestimation of SaO2 levels.
When a patient is cyanotic and methemoglobinemia is suspected, a co-oximeter capable of measuring the absorbance of greater than 2 wavelengths of light is necessary to determine fractional oxygen saturation and MetHb levels.
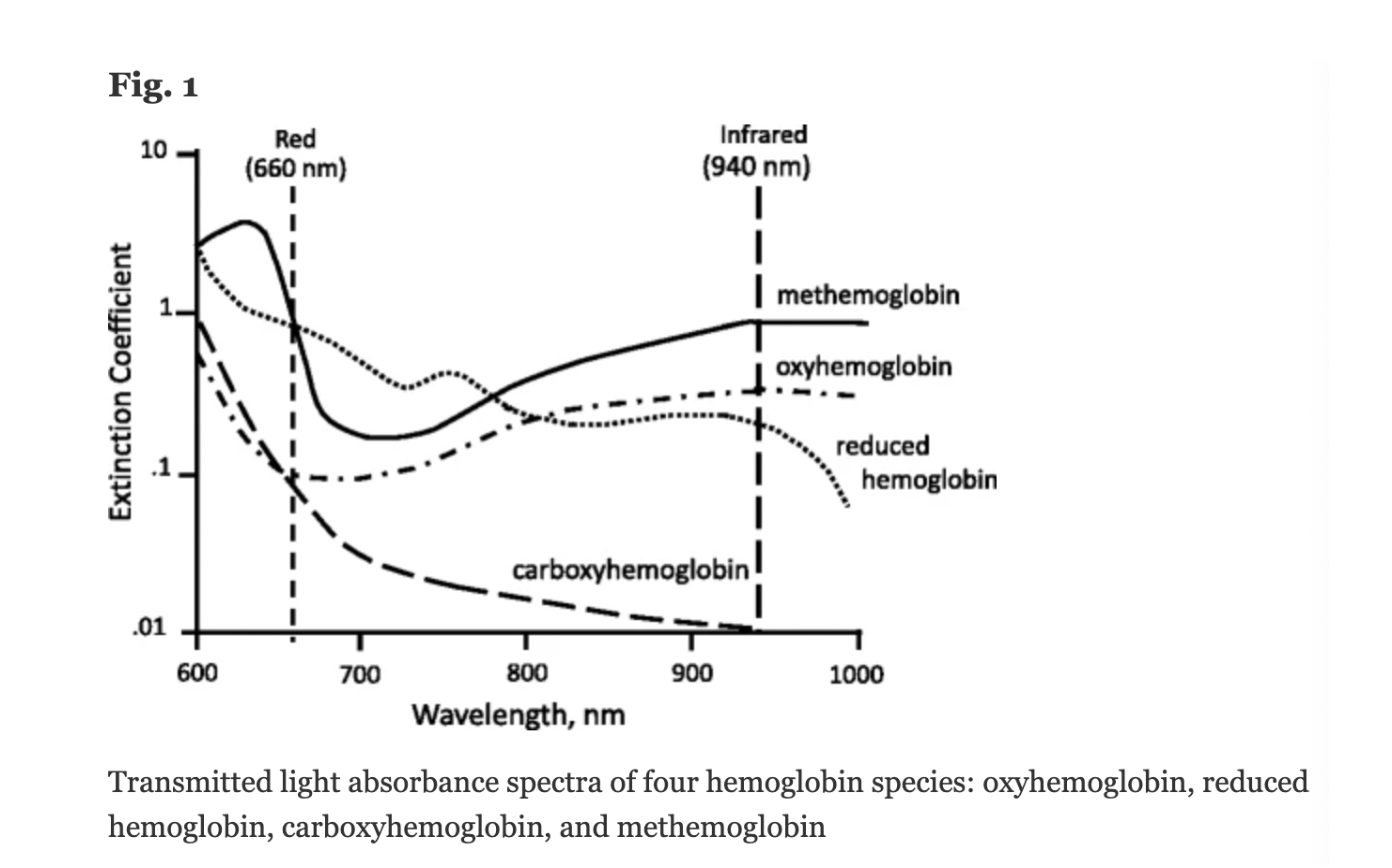
Figure: Jubran, Critical Care, 2015
Keywords: Dyshemoglobin, Methemoglobin, Methemoglobinemia,
References: Jubran, Critical Care, 2015 Chan et al, Respiratory Medicine 2013